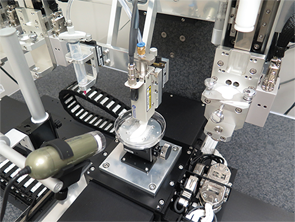
The Integrated Tissue-Organ Printing system at work printing a jawbone structure.
Courtesy of Wake Forest University in North Carolina
When Abby Paterson, PhD, started her doctoral work in product design and technology at Loughborough University in the United Kingdom in 2009, she says 3D printing was little known by clinicians or the general public. Now, the technology is seemingly everywhere.
For Dr. Paterson, the advancing science has led to a promising project focused on reimagining wrist splints for patients with rheumatoid arthritis (RA). For rheumatologists, Dr. Paterson’s work is just one of many examples of how 3D printing is reshaping both external devices and internal joint replacements. Bioprinting aimed at regenerating cells and tissues, while earlier in the development pipeline, is also rapidly progressing.
Frank Rybicki, MD, PhD, professor, chair and chief of radiology at the University of Ottawa in Canada and editor in chief of the new journal 3D Printing in Medicine, says he’s seeing the biggest burst of printing-related activity in cardiovascular, trauma and orthopedic–rheumatologic research.
Rheumatology applications are receiving an extra boost from new printing materials that can more closely replicate the varied properties of joints, improved printing resolution and a boom in 3D-printed products aimed at consumers. “Printing things that are worn on the body, whether they be for glamour or for function or for healing, is a large field that’s just growing exponentially,” Dr. Rybicki says. That’s good news for rheumatologists, therapists and patients hoping for an upgrade of outdated splint fabrication techniques.
Blueprint for a Better Splint
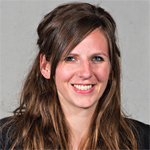
Dr. Paterson
Dr. Paterson, now a 3D-printing specialist and lecturer in product design at Loughborough University, says traditional wrist splints for patients with RA are typically made of plastic. They’re often poorly ventilated and usually difficult to clean, especially if they contain a padded lining where perspiration can collect. Therapists have told her that some patients avoid social settings because their splints are too unsightly and smelly or telegraph their disability to others. Worse, splints that slide snugly over enlarged joints or rub against the skin can add to a patient’s discomfort.
As a result, physical and occupational therapists often struggle to keep their splint-wearing patients in compliance. For her design work, Dr. Paterson sought input from 10 therapists throughout the UK, who assured her there was a big need for a better solution.
To construct a 3D-printed alternative, Dr. Paterson first uses 3D laser scanning or other optical scanning methods to yield a high-resolution image of a patient’s arm in the correct position (her group has also been experimenting with low-cost scanners that could be used in clinics). With the dimensions in hand, a computer-aided design program can convert the scanning data into a 3D model and create a splint design. To help healthcare professionals navigate what can be a steep learning curve, Dr. Paterson’s team is working with hand therapists to develop a clinician-friendly software program that can streamline the splint design process.
‘It will be very difficult to do bioprinting for joints, but it’s not far-fetched that we’ll be able to bioprint complex structures in the future.’ —Dr. Rybicki
Starting with plastics known as acrylic-based photopolymer resins, Dr. Paterson has designed a wide range of printable wrist splints that are moving toward commercialization. One, built on a multi-material, multi-color machine called Connex3, features a multi-material latticework design with a clamshell hinge that lets a patient easily slide an arm into the splint and avoid aggravating tender joints, muscles and tendons. The splint fastens along the top of the forearm, while soft areas cushion bony areas of the wrist, such as the ulnar styloid. The multi-colored splint is also designed to be attractive, personalizable, sporty and breathable.
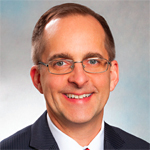
Dr. Rybicki
A less rigid mesh splint is made through a separate 3D-printing process called laser sintering and could be a boon for older patients and others with sensitive skin. Part of the splint is printed textile that serves as an easily opened hinge. “The material is also very strong, and if it gets dirty, it can be placed in a dishwasher for cleaning,” Dr. Paterson says.
By incorporating multiple materials into the design, a splint could provide extra cushioning over rheumatoid nodules and painful joints. “Rubber-like material could also be applied to expand and contract with joints, which are liable to fluctuating swelling, too,” Dr. Paterson says. “All these aspects are currently not possible with traditional splinting without changing the physical shape of the splint, which can sometimes make them look cumbersome. So 3D printing can offer some exciting new avenues to explore.”
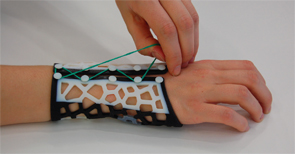
Dr. Paterson’s lab is reimagining wrist splints for patients with rheumatoid arthritis.
Courtesy of dr. abby paterson
Customizable splints could even accommodate add-ons for daily activities. Auto manufacturer BMW outfitted assembly workers at its Munich, Germany, plant with customized 3D-printed gloves with a thumb support to reduce the incidence of repetitive stress injuries. In the same vein, Dr. Paterson says, designers could create specialized splints that allow patients to perform tasks while maximizing their support. Although her team is focusing on the wrist, she says the same design concept could be applied to other joints throughout the body, such as the shoulder, knee or ankle.
Angie Dickerson-Schnatz, an orthopedic-certified specialist and a certified hand therapist at the Philadelphia Hand Center in Glen Mills, Pa., agrees that such technology, if cost effective, could make a big difference in improving patient compliance around splints that are worn for long periods of time. “If it’s more uncomfortable and difficult to put the thing on than it is to just live with your arthritis, then you never wear it,” she says.
For hip & knee joint replacements, 3D-printing advocates see a clearer future of increasingly customized & precisely fitting implants on demand.
Cleanliness is another big concern. “If somebody has a splint that they have to wear all the time and it stinks, nobody wants to be friends with that person anymore,” Ms. Dickerson-Schnatz says. “You’re eating with your hand, and it smells like a dead ’possum.”
A lightweight, breathable, aesthetic and easily cleaned splint that snaps into place around a patient’s arm and allows for customized add-ons would be a big step forward. “Certainly for this practice, it’s something that we would definitely consider,” she says.
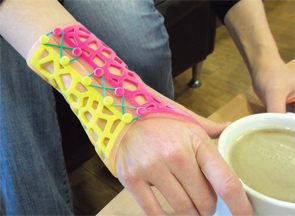
This splint’s multi-material latticework design with a clamshell hinge lets a patient easily slide an arm into the splint and avoid aggravating tender joints, muscles and tendons.
Courtesy of dr. abby paterson
In Italy, the +Lab at Polytechnic University of Milan has created a separate range of innovative 3D-printed materials through its +TUO (more yours) Project. The effort has yielded a half-dozen small tools aimed at simplifying everyday activities for patients with rheumatic diseases.
One helps patients open plastic bottle caps, while another helps turn a key in a lock. There’s a spoon holder, computer mouse support, cutting board setup and set of kitchen gloves with an improved grip. Ms. Dickerson-Schnatz says such devices could be beneficial if they’re affordable and modifiable based on individual patient needs. “Those are the kinds of things that would be nice to customize to make them more functional,” she says.
Makeover for Artificial Joints
Rheumatologists and other clinicians have expressed similar sentiments about exploiting 3D printing’s potential to produce more personalized joint replacements. Printing joints is trickier than bones because the soft-tissue components show up less well on CTs, and the printing process requires more fine-tuning. Nevertheless, Dr. Rybicki says, “There’s a growing interest in 3D printing of joints and joint structures, and there are a lot of possibilities there.”
Newly available printing materials, for example, can provide better representation of soft or cartilaginous tissues, allowing researchers to build more complex models that better approximate the physical and mechanical properties of joint structures. Scientists are also modifying diagnostic MRIs to provide higher spatial resolution in all three dimensions—a necessity for any 3D printing and modeling work.
Because titanium’s stiffness matches that of bone, the lightweight but durable metal has long been popular as a joint implant material. In September 2015, China’s Food and Drug Administration formally approved a 3D-printed, titanium alloy-based acetabular cup prosthesis for the hip joint. Meanwhile, British firms Metalysis and TWI have joined forces to develop a 3D-printing process akin to what’s used for manufacturing automotive parts. Instead of titanium, the process uses a bio-inert powder form of the metal tantalum as the “ink” to create layers of a latticework grid, and then uses lasers to fuse the metal together into a strong structure. If proved, the process could drive down costs and yield more personalized joint replacements instead of limiting patients to available sizes.
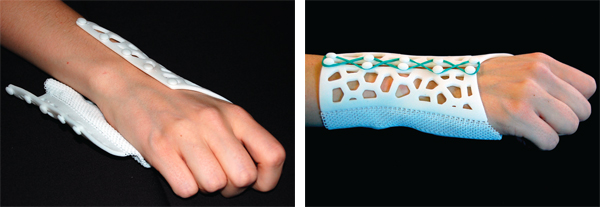
This additive, manufactured textile splint could be a boon for older patients and others with sensitive skin. Part of the mesh splint is printed textile that serves as an easily opened hinge.
Courtesy of dr. abby paterson
Other applications have raised intriguing possibilities for educating both patients and doctors. For example, medical consultant Axel Hueber, MD, PhD, and clinical research fellow Arnd Kleyer, MD, both in the rheumatology department at the Friedrich-Alexander University Erlangen-Nürnberg in Germany, are using high-resolution CT imaging to create detailed 3D-printed models of arthritic joints. Specifically, they and colleagues have used a cement-like powder to print life-size and magnified models of damaged metacarpal heads from the hands of patients with RA.
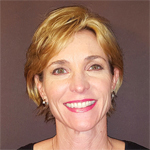
Ms. Dickerson-Schnatz
With a print resolution up to 80 microns, the process has allowed the research team to precisely localize and measure bone erosions and other damage, such as significant reductions in the periarticular bone’s trabecular network.
Dr. Kleyer says the researchers initially saw the technology as a way to create 3D-printed wrist joint replacements, and they printed titanium joints as a proof of concept. Orthopedists, however, cautioned them that the wrist joint’s complicated anatomy and physiology would make artificial replacements exceedingly difficult in the near future. Instead, the German rheumatologists are assessing whether their technique can help improve patient education, monitor damage and improve site-directed injections.
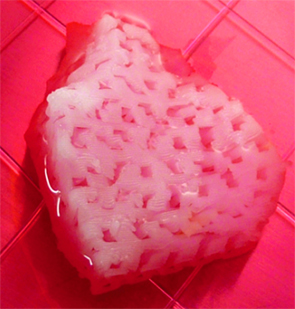
A 3D computer-aided design program was used to make a model of a jawbone segment that needed to be replaced. This image shows the tailor-made, bioprinted jawbone structure.
Courtesy of Wake Forest University in North Carolina; Left:
For all three applications, printing an enlarged arthritic joint that more clearly shows the damage could be especially valuable. The increased understanding could help patients better visualize the progressive destruction of their joints and give them an idea of what future stages of disease might look like under various scenarios. Although feedback has been positive so far, Dr. Hueber says the team is continuing to analyze whether patients find the 3D-printed models valuable. “We are going to do this study to understand if this is something the patient actually wants to see,” he says, “or if it’s something like smokers who don’t want to see black lung.”
If it’s the former, the better-informed patients might adhere more closely to therapy and medication regimens. As for clinician education, other researchers have already created 3D-printed horse joints to help veterinarians learn and practice joint injections. In the same way, Dr. Hueber says, a 1:1 model of an arthritic hand might be a valuable educational and training tool for rheumatologists and orthopedists.
For hip and knee joint replacements, 3D-printing advocates see a clearer future of increasingly customized and precisely fitting implants on demand. All of them must still contend with a major challenge, however: any mismatch in stiffness between implant and bone can produce a stress-shielding effect that leads to calcification and implant failure. Even implants that closely match bone stiffness may last only 20 years at most—one reason why multiple researchers are also exploring more permanent joint replacement solutions for younger patients through regenerative medicine.
New Potential for Natural Replacements
Anthony Atala, MD, director of the Wake Forest Institute for Regenerative Medicine and the W. Boyce Professor and Chair of Urology at Wake Forest University in North Carolina, says 3D printing is furthering regenerative medicine’s goal of restoring functional tissues, organs and joints. “The way you do that is by taking a small biopsy of the patient, and once you have those cells, you’re able to put the tissues together using different scaffold systems,” he says. Using a patient’s own cells can help avoid transplant rejection, but the technique’s scope has been limited by the hand-assembled scaffolding.
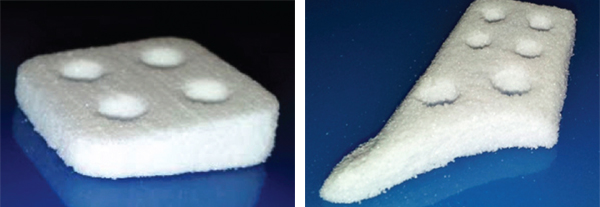
In Dr. Toyserkani’s lab, the features of three printing technologies have been combined to print a calcium polyphosphate scaffold with variable pore distribution to create biodegradable femur implants.
The Friedrich-Alexander University Erlangen-Nürnberg in Germany
3D printing can help automate the process and yield highly reproducible and scalable structures fashioned with biodegradable polymers. The challenge, of course, is printing living cells in a way that preserves their viability while creating a precise pattern instead of a poorly resolved blob. A fine nozzle on Dr. Atala’s Integrated Tissue and Organ Printing (ITOP) system helps provide precise placement. The right cell consistency is also critical. “You want them to go through the nozzle as a liquid, but then you want them to hit the surface as a semi-soft hydrogel so that they retain their shape,” he says.
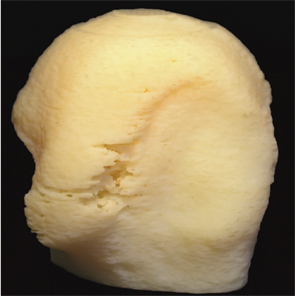
Drs. Hueber and Kleyer, of the Friedrich-Alexander University Erlangen-Nürnberg in Germany, are using high-resolution CT imaging and a cement-like powder to create detailed 3D-printed models of damaged metacarpal heads from the hands of patients with RA. The technique can help improve patient education, monitor damage and improve site-directed injections.
Courtesy of University of Waterloo in Canada
Finally, the resulting 3D structures need to allow for cell and tissue growth. Dr. Atala’s lab has helped solve this issue, as well, by creating microchannels that provide conduits for nutrients and oxygen to reach the central portion of a printed construct and help keep the cells alive. So far, his group has used the technique to successfully print skeletal muscle tissue, more elastic cartilage and stronger bone tissue, and the lab is now conducting preclinical studies as a precursor to eventual clinical applications.1
Proving the method’s safety and efficacy in humans will be essential, but the success so far at least opens the door to regenerating the complex mixed-tissue architecture of joints. “It will be very difficult to do bioprinting for joints, but it’s not far-fetched that we’ll be able to bioprint complex structures in the future,” Dr. Rybicki notes.
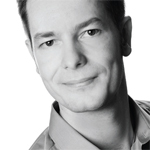
Dr. Hueber
Newer materials and innovative techniques could significantly speed up the timeline. Ehsan Toyserkani, PEng, professor and university research chair in additive manufacturing at the University of Waterloo in Ontario, Canada, has developed a separate 3D-printing process that can make complex scaffolds out of a biodegradable powder called calcium polyphosphate. “It worked perfectly, actually,” he says.
To achieve their goal of personalized 3D-printed joints, the researchers hatched a plan to use the scaffold as the temporary support structure for a patient’s own stem cells. The cells could be coaxed into growing cartilage, bone and other tissue, thereby eventually replacing a damaged joint. The team, however, realized that the printed structure would need to contain a variable pore distribution system to account for the wildly varying speeds at which osteoblasts and cartilage grow in a porous media.
“That was a challenge, because 3D printing can make good parts with a complex shape, but we cannot really control the internal properties when it comes to macro- and micro-level interconnected porosities,” Dr. Toyserkani says. “That’s why we started to think outside the box, and we developed a new 3D printer.” His retooled update has combined the features of three printing technologies to create a calcium polyphosphate scaffold with variable pore distribution. And by printing the scaffold in a specific orientation, he and collaborators have been able to significantly increase the implant’s mechanical strength.2
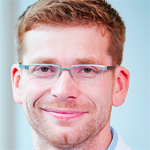
Dr. Kleyer
Encouraged by their results thus far, Dr. Toyserkani and colleagues at the University of Toronto and Mount Sinai Hospital in Ontario are working toward a type of complete knee replacement. As with many other promising techniques, the printed version will have to pass regulatory muster, but he sees plenty of potential upside. “My expectation is that in five years’ time, 3D printing would be a mandatory piece of equipment for many orthopedic laboratories, to really make implants on demand from biodegradable materials,” he says.
Jeremy Mao, DDS, PhD, the Edwin S. Robinson Professor of Dentistry and a professor of orthopedic surgery and biomedical engineering at Columbia University in New York, also has been using 3D printing to regenerate a variety of body tissues. 3D printing has great potential in orthopedics, because it can handle the challenges of recreating structural tissues. “Not only do you need to regenerate the tissues’ function, but also they need to have certain shapes and dimensions that fit the patients’ needs,” he says.
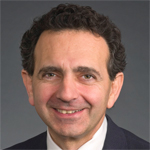
Dr. Atala
For patients suffering from osteoarthritis and rheumatoid arthritis, Dr. Mao says, “that’s where I think 3D printing becomes really vital for the regeneration of musculoskeletal structures.” He has developed his own technique to coax a patient’s endogenous stem cells into regrowing tissue. In 2010, his lab used the method to regenerate cartilage in rabbits and in 2014, he successfully replaced the meniscus in a sheep.3
Dr. Mao’s 3D-printing process creates a scaffolding of biocompatible and bioresorbable materials and provides the migration and differentiation cues needed to recruit endogenous stem cells to the structure in order to initiate tissue regeneration. One biomaterial, a biodegradable type of polyester known as polycaprolactone, is already FDA approved. “So our innovation was to use this material that was previously approved for a surgical suture and then print it into the shape and dimension of cartilage structures and bone structures,” Dr. Mao says.
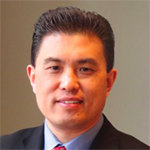
Dr. Mao
As with other regenerative medicine approaches, Dr. Mao says demonstrating the safety, efficacy and durability of his technique and materials will require diligent testing in animal models and then in human clinical trials. He notes that the safety bar would be even higher for cells removed and then transplanted back into a patient, however, because cultured cells can become contaminated or transform into tumors.
A Bright Future
Despite the remaining challenges, 3D printing is advancing quickly for both internal and external rheumatology applications due to more sophisticated materials, faster and cheaper printers and improving knowledge and skillsets. And amid the recent flurry of activity, Dr. Paterson believes there’s plenty more to come. “It’s going to be a really exciting area to follow,” she says.
Bryn Nelson, PhD, is a medical journalist based in Seattle.
References
- Kang, H-W, Lee SJ, Ko IK, et al. A 3D bioprinting system to produce human-scale tissue constructs with structural integrity. Nat. Biotech. 2016 Feb 15;34:312–319.
- Shanjania Y, Hub Y, Pilliarb RM, Toyserkani E. Mechanical characteristics of solid-freeform-fabricated porous calcium polyphosphate structures with oriented stacked layers. Acta Biomater. 2011 Apr;7(4):1788–1796.
- Lee CH, Rodeo SA, Fortier LA, et al. Protein-releasing polymeric scaffolds induce fibrochondrocytic differentiation of endogenous cells for knee meniscus regeneration in sheep. Sci Transl Med. 2104 Dec 10;6(266):266ra171.