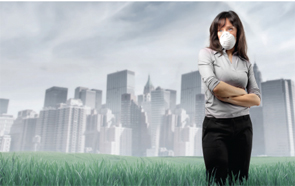
Image Credit: Ollyy/shutterstock.com
Interactions between an individual’s genetic background and their exposure to environmental factors are thought to result in a cascade of immune reactions, ultimately leading to the development of autoimmune diseases such as rheumatoid arthritis (RA), juvenile idiopathic arthritis (JIA), systemic lupus erythematosus (SLE) and juvenile dermatomyositis.1,2 For example, an environmental factor that conclusively affects susceptibility to the development of RA is smoking, but with risk magnified by the presence and number of copies of shared epitope.3,4
Air pollution is a recognized risk factor for respiratory and cardiovascular disease, but has also been associated with immune-mediated diseases, such as inflammatory bowel disease and multiple sclerosis.5,6 Air pollution may mediate its effect on autoimmune disease by directly stimulating an inflammatory response or indirectly through alterations in the microbiome.7-9 Thus, air pollution has been hypothesized to influence the development of rheumatic diseases. In this review, we summarize sources of air pollution, how levels can be measured and what evidence exists for associations between rheumatic disease development and exposure to air pollution.
Sources of Air Pollution
Air pollution comprises a complex mixture of particles and gases from a variety of industrial and private sources. Fossil fuel use is the main source of emission of airborne pollutants in developed countries. In general, exposure to air pollution can result in activation of the immune response; an early study demonstrated measurable differences in immunoglobulins, complement components, haptoglobin and a-1-glycoprotein between a female population exposed to higher compared to lower atmospheric pollution.10
Particulate matter (PM) <2.5µm in size (PM2.5) has been the focus of most of the published literature on air pollution and health. PM air pollution is a mixture of solid, liquid, or solid and liquid particles suspended in air that can travel long distances from remote sources or be concentrated by weather-related factors (e.g., winter inversions). It is largely composed of ammonium sulfate and ammonium nitrate derived from diesel fuel combustion and products of motor vehicle gas combustion and coal-powered power plants.11,12 PM2.5 particles can penetrate the gas-exchange region of the lung, creating oxidative stress and triggering activation of macrophages and epithelial cells in the alveolar tissues, ultimately resulting in an inflammatory response and secretion of pro-inflammatory cytokines.11,13
Other measured pollutants include particulate matter <10µm in size (PM10), sulfur dioxide (SO2) and nitrogen dioxide (NO2). PM10 particles are larger and are, therefore, less likely to be carried from distant sources or penetrate the trachea-bronchial tree. SO2 is formed during petroleum refining or cement manufacturing and also from coal-fired power plants, locomotives, ships and some non-road diesel-burning equipment.14
NO2, also known as nitrogen peroxide, is a reddish-brown gas at room temperature and has numerous industrial applications, such as serving as a nitrating agent for explosives and as an oxidizing agent in rocket propulsion.15 Potential occupation exposures to NO2 include fuel combustion in motor vehicles and stationary power plants, etching of metal with nitric acid, as well as acetylene and electric cutting and welding.15 In agricultural settings, fermentation of recently stored agricultural silage can also produce NO2. Motor vehicle traffic is a common source of NO2 exposure in the general population, and indoor sources include poorly vented gas ranges, and kerosene or gas space heaters.15 NO2 is readily absorbed and nearly all inhaled compound is absorbed through the tracheo-bronchial tree.12,15
Less frequently assessed are exposures to black carbon and carbon monoxide (CO), both produced by the incomplete combustion of hydrocarbon materials, such as during the operation of internal combustion engines.16,17
Ozone is also a pollutant, produced when nitrogen oxides (NOx) and volatile organic compounds (VOCs) interact under the action of sunlight.18 NOx are also generated by fossil fuel combustion, such as from motor vehicle engines; power plants; industrial plants; and boilers; VOC sources are motor vehicle emissions, gasoline vapors and chemical solvents.18 Ozone creation, destruction and dispersion are highly dependent on temperature, wind speed and direction, solar radiation and humidity.18 High concentrations of ozone are observed over large cities and metropolitan areas during warm months.18
Measuring Air Pollution Exposure in Research Studies
Most metropolitan cities have dedicated automated fixed-site continuous monitoring stations that collect hourly mean levels of criteria air pollutants (i.e., O3, NO2, SO2, CO, PM10 and PM2.5). The hourly data can be averaged to obtain daily estimates for the entire city and provide air pollution data for temporal analyses; however, these estimates are often not precise across geographic regions.
Air pollution is a recognized risk factor for respiratory & cardiovascular disease, but has also been associated with immune-mediated diseases, such as inflammatory bowel disease & multiple sclerosis.
Land-use regression (LUR) or inverse density weighting is applied to estimate pollution exposure at a given geographical point, typically an individual’s location of residence (determined by postal code). LUR models use Geographic Information System (GIS) to estimate air pollutant exposure through a combination of land use, traffic, population density, physical geography and meteorology across an area and predict concentrations at a defined site.19,20
Inverse distance weighting uses the general principle of interpolation, where values of a given variable are estimated and derived from a known and measured value at another point (e.g., a monitoring station), and sources farther from the reference point will have a lower impact of the pollution.21
Another way to measure air pollution across geographic locations is remote sensing through satellite imaging. The advantage of remote sensing is its broad geographic coverage, including remote areas without dedicated pollution monitors; however, maps covering geographic areas are large (typically 10×10 km grids), which reduce the resolution of geocoding individuals living in these areas.
Evidence for an Association Between Air Pollution Exposure & the Development of Rheumatic Diseases
Rheumatoid Arthritis
In the prospective Nurses’ Health Study, after adjustment for multiple confounders, there was no evidence for an increased risk of RA by exposure to NO2 (HR 0.92, 95%CI 0.85–1.00), SO2 (HR 0.99, 95%CI 0.90–1.09), PM2.5 (HR 0.94, 95%CI 0.86–1.04) nor PM10 (HR 0.92, 95%CI 0.85–0.99).22
Two case-control studies have also been reported. A study using an administrative health dataset from Canada also failed to show an association between air pollution and RA development and in fact, had indication of a protective effect from exposure. The respective odds ratios (ORs) for incident RA and pollutants after adjustment for age, sex and neighborhood socioeconomic status were: NO2 (OR 0.90, 95%CI 0.85–0.96), PM2.5 (OR 0.92, 95%CI 0.87–0.98), PM10 (OR 0.91, 95%CI 0.86–0.96) and SO2 (OR 0.88, 95%CI 0.82–0.93).23
The inverse association is puzzling and potentially points to methodological issues in identifying patients from administrative data and assigning exposures.
This study also assessed risk relative to exposures for nitrous oxide (NO), black carbon, ozone and CO, with all ORs below 1.00—except for ozone (OR 1.07, 95%CI 0.98–1.16).
In the Swedish Epidemiological Investigation of Rheumatoid Arthritis (EIRA) study, no definite association was detected between incident RA and NO2 (OR 0.98, 95%CI 0.90–1.07), SO2 (OR 1.01, 95%CI 0.93–1.09) nor PM10 (OR 0.96, 95%CI 0.88–1.04), when correcting for age, sex, smoking status and education.24
Juvenile Idiopathic Arthritis
Increased concentrations of PM2.5 related to stagnant air conditions in a population from Utah was associated with an elevated relative risk (RR) of JIA onset in preschool aged children (RR 1.60, 95%CI 1.00–2.54), but not older children.25 In a subsequent abstract by the same author, using a metropolitan North American cohort consisting of residents from Boston, Philadelphia, Atlanta, Cincinnati and Toronto, no significant association between PM2.5 exposure and a risk of systemic JIA onset was found, although when pooled with the cases from Utah, the significant association for younger children was again demonstrated (RR 1.76, 95%CI 1.07–2.89 per 10 µg/m3 increase in the three-day lagged moving average of PM2.5).26
Of interest, is retrospective research from São Paolo, Brazil. A case-control study comprising mothers of 21 JIA and 40 control subjects, matched by age and sex, completed a questionnaire that assessed demographic data and environmental inhalation exposure during pregnancy, and estimated daily concentrations of PM10, SO2, NO2, ozone and CO in the pre-gestational and gestational periods. Intriguing was that although they found smoking to have a significant effect on increasing JIA incidence, as might be expected, they also found that exposure to long-distance industrial activities or gas stations could, in fact, be a protective factor.27
Systemic Autoimmune Rheumatic Diseases (SARDs)
All of the studies on associations between PM2.5 air pollution exposure and the development of SARDs (systemic lupus erythematosus, Sjögren’s syndrome, scleroderma, polymyositis, dermatomyositis or undifferentiated connective tissue disease) come from Canada. Population-based administrative data from two provinces, Alberta and Quebec, were used in one study, and significant associations found. In Quebec residents, the adjusted OR for being a case of SARDs was increased by PM2.5 levels (OR 1.05 per µg/m3, 95%CI 1.05–1.06). In Alberta, First Nations populations were particularly susceptible, with an adjusted OR of 1.38 (95%CI 1.14–1.68) per µg/m3.28 In the city of Calgary, the odds of being a SARDs case increased with PM2.5 levels (OR 1.10 per µg/m3, 95%CI 1.01–1.22), again with First Nations being at increased risk compared to non-First Nations residents, although an association with NO2 could not be clearly detected.29
Vasculitis & Juvenile Dermatomyositis
In a U.K. study, no evidence for spatial clustering, PM10 exposure and ANCA-associated vasculitis was found.30 Similarly, in a North American study, no link between PM2.5 and Kawasaki disease was found, after controlling for time trends, seasonality, month and weekday.31
Using multivariate analysis controlling for smoking, occupational exposure, home distance to sources of inhaled pollutants, seasonality and traffic exposure, a Brazilian study showed a significant association between CO exposure in the third trimester and the subsequent development of juvenile dermatomyositis (OR 12.2, 95%CI 1.3–116.0).32
Effect of Air Pollution on Disease Activity in Established Rheumatic Disease
Data are sparse on this topic. Canadian data have shown that SLE disease activity is influenced by exposure to PM2.5. Hourly PM2.5 concentrations were taken from fixed-site stations, and levels were averaged over 24 hours for the day preceding clinical review. Higher exposure was associated with levels of anti-dsDNA antibodies (adjusted OR 1.34, 95%CI 1.02–1.77).33
A Brazilian study demonstrated a delayed association between SO2 exposure and hospital admissions due to pediatric rheumatic diseases. There was an increase in the number of admissions by 1.98% (95%CI 0.25–3.69), and the maximum effect was noted 16 days after the exposure.34
Knowledge Gaps
At this time, there is no definitive association determined for any air pollutant in any rheumatic disease. More data are needed to elucidate the progression from pollution-related immune stimulation, the formation of antibodies and the ultimate progression to clinically apparent disease, as well as the effect of air pollution on disease phenotype, prognosis and activity. Another outstanding issue that needs to be addressed by future research is the potential for differential toxicity between source types.
As for the risk associated with the timing of exposure, fetal and childhood exposure may be important. Several JIA and juvenile dermatomyositis studies demonstrate a heightened risk related to the gestational period and in children <5.5 years of age. The potential impact of ethnicity and underlying genetic background is evidenced by the Canadian data, showing an association of SARDs development particularly in First Nations patients.
There are significant issues with exposure definition in this area of research. Determination of exposure is typically based on place of residence, rather than work or commute exposures, or even accounting for duration of exposure. This simplification may introduce substantial exposure misclassification and may explain the large number of null results seen in the studies published to date.35 Further, the variable latency of subclinical and clinical rheumatic disease can make determination of a clear link difficult. The ability to investigate personal exposures will be a significant advance in this field, while further accounting for the myriad potential genetic–environmental interactions that may explain disease development and onset, and improvements in disease detection and diagnosis.
Conclusion
To date, the evidence for an association between air pollutant exposure and the risk of developing rheumatoid arthritis or vasculitis is unclear; however, the odds of developing SARDs with exposure to PM2.5 do appear increased, particularly in the First Nations populations in Canada. Studies of exposure to air pollution in the gestational period or early childhood suggest an increased risk for developing JIA and juvenile dermatomyositis with multiple pollutants.
At this time, there is no definitive association determined for any air pollutant in any rheumatic disease.
Numerous challenges exist with regard to establishing a link between the exposure to air pollution and development of rheumatic disease, in particular the measurement of exposure, the contribution of different sources of air pollution and timing of exposure. Further refinements in methodology will be beneficial to improving estimates and truly determining whether air pollution does in fact play an important role in the development of rheumatic disease.
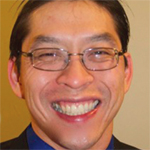
Dr. Sun
Gavin R. Sun, MD, is a third-year internal medicine resident with an interest in rheumatology. He completed medical school at the University of the Witwatersrand in Johannesburg, South Africa in 2006. Upon completion of his internship and community service, he emigrated to Canada in 2010. He previously practiced as a rural family physician in Vulcan, Alberta, between 2010 and 2012 before returning to residency at the University of Calgary.
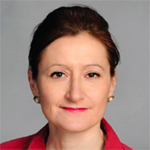
Dr. Bernatsky
Sasha Bernatsky, MD, is a rheumatologist and epidemiologist at McGill University. She specializes in the use of administrative datasets to study the epidemiology and outcomes of rheumatic diseases.
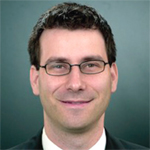
Dr. Kaplan
Gilaad G. Kaplan, MD, is a gastroenterologist and an associate professor in the Department of Medicine and Department of Community Health Sciences at the Cumming School of Medicine at the University of Calgary. His research expertise is applied to study the relationships between inflammatory bowel diseases and environmental exposures.
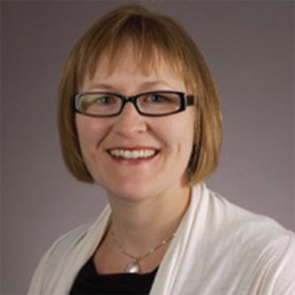
Dr. Barnabe
Cheryl Barnabe, MD, is a rheumatologist and assistant professor in the Department of Medicine and Department of Community Health Sciences at the Cumming School of Medicine at the University of Calgary. She is trained in clinical epidemiology and has a primary interest in rheumatoid arthritis.
References
- Gibofsky A. Overview of epidemiology, pathophysiology, and diagnosis of rheumatoid arthritis. Am J Manag Care. 2012 Dec;
- 18(13 Suppl):S295–S302.
- Widdifield J, Paterson JM, Bernatsky S, et al. The epidemiology of rheumatoid arthritis in Ontario, Canada. Arthritis Rheumatol. 2014 Apr;66(4):786–793.
- Sugiyama D, Nishimura K, Tamaki K, et al. Impact of smoking as a risk factor for developing rheumatoid arthritis: A meta-analysis of observational studies. Ann Rheum Dis. 2010 Jan;69(1):70–81.
- Padyukov L, Silva C, Stolt P, et al. A gene-environment interaction between smoking and shared epitope genes in HLA-DR provides a high risk of seropositive rheumatoid arthritis. Arthritis Rheum. 2004 Oct;50(10):3085–3092.
- Kaplan GG, Hubbard J, Korzenik J, et al. The inflammatory bowel diseases and ambient air pollution: A novel association. Am J Gastroenterol. 2010 Nov;105(11):2412–2419.
- Oikonen M, Laaksonen M, Laippala P, et al. Ambient air quality and occurrence of multiple sclerosis relapse. Neuroepidemiology. 2003 Jan–Feb;22(1):95–99.
- Tornqvist H, Mills NL, Gonzalez M, et al. Persistent endothelial dysfunction in humans after diesel exhaust inhalation. Am J Respir Crit Care Med. 2007 Aug 15;176(4):395–400.
- Salim SY, Kaplan GG, Madsen KL. Air pollution effects on the gut microbiota: A link between exposure and inflammatory disease. Gut Microbes. 2014 Mar–Apr;5(2):215–219.
- Kish L, Hotte N, Kaplan GG, et al. Environmental particulate matter induces murine intestinal inflammatory responses and alters the gut microbiome. PLoS One. 2013 Apr 24;8(4):e62220.
- Stiller-Winkler R, Idel H, Leng G, et al. Influence of air pollution on humoral immune response. J Clin Epidemiol. 1996 May;49(5):527–534.
- Brunekreef B, Holgate ST. Air pollution and health. Lancet. 2002 Oct 19;360(9341):1233–1242.
- Seaton A, MacNee W, Donaldson K, et al. Particulate air pollution and acute health effects. Lancet. 1995 Jan 21;345(8943):176–178.
- Kelly FJ, Fussell JC. Linking ambient particulate matter pollution effects with oxidative biology and immune responses. Ann N Y Acad Sci. 2015 Mar;1340:84–94.
- Chen TM, Gokhale J, Shofer S, et al. Outdoor air pollution: Nitrogen dioxide, sulfur dioxide, and carbon monoxide health effects. Am J Med Sci. 2007 Apr;333(4):249–256.
- Luttrell WE. Nitrogen dioxide. J Chem Health Saf. 2014 Mar;21(2).
- Koelmans AA, Jonker MT, Cornelissen G, et al. Black carbon: The reverse of its dark side. Chemosphere. 2006 Apr;63(3):365–377.
- Von Burg R. Carbon monoxide. J Appl Toxicol. 1999 Sep–Oct;19(5):379–386.
- Mustafa YA, Mohammed SJ. Measurement of ground level ozone at different locations. Am J Environ Sci. 2012;8(3):311–321.
- Henderson SB, Beckerman B, Jerrett M, et al. Application of land use regression to estimate long-term concentrations of traffic-related nitrogen oxides and fine particulate matter. Environ Sci Technol. 2007 Apr 1;41(7):2422–2428.
- Bellander T, Berglind N, Gustavsson P, et al. Using geographic information systems to assess individual historical exposure to air pollution from traffic and house heating in Stockholm. Environ Health Perspect. 2001 Jun;109(6):633–639.
- de Mesnard L. Pollution models and inverse distance weighting: Some critical remarks. Comput Geosci. 2013 Mar;52:459–469.
- Hart JE, Kallberg H, Laden F, et al. Ambient air pollution exposures and risk of rheumatoid arthritis. Arthritis Care Res (Hoboken). 2013Jul;65(7):1190–1196.
- De Roos AJ, Koehoorn M, Tamburic L, et al. Proximity to traffic, ambient air pollution, and community noise in relation to incident rheumatoid arthritis. Environ Health Persp. 2014 Oct;122(10):1075–1080.
- Hart JE, Kallberg H, Laden F, et al. Ambient air pollution exposures and risk of rheumatoid arthritis: Results from the Swedish EIRA case-control study. Ann Rheum Dis. 2013 Jun;72(6):888–894.
- Zeft AS, Prahalad S, Lefevre S, et al. Juvenile idiopathic arthritis and exposure to fine particulate air pollution. Clin Exp Rheumatol. 2009 Sep–Oct;27(5):877–884.
- Zeft A, Prahalad S, Schneider R, et al. Systemic juvenile idiopathic arthritis and exposure to fine particulate air pollution. Abstract 319. Arthritis Rheumatol. 2014 Oct;66(10 Suppl):S136.
- Franca CMP, Sallum AM, Silva CAA, et al. Risk factors for juvenile idiopathic arthritis: Exposure to tobacco and environmental factors during and before pregnancy. Pediatric Rheumatology. 2014;12(Suppl1):27.
- Bernatsky S, Smargiassi A, Barnabe C, et al. Fine particulate air pollution and systemic autoimmune rheumatic disease in two Canadian provinces. Abstract 2015. Arthritis Rheumatol. 2014 Oct;66(10 Suppl):S884–S885.
- Bernatsky S, Smargiassi A, Johnson M, et al. Fine particulate air pollution, nitrogen dioxide, and systemic auto-immune rheumatic disease in Calgary, Alberta. Environ Res. 2015;140:474–478.
- Watts R, Nelson D, Mooney J, et al. Is there spatial clustering of ANCA-associated vasculitis in the UK? Abstract OP43. Rheumatology (Oxford). 2009 Apr;48(Suppl 1):i16.
- Zeft AS, Burns JC, Yeung RS, et al. Kawasaki disease and exposure to fine particulate air pollution. Ann Rheum Dis. 2015;74(A47).
- Orione MA, Silva CA, Sallum AM, Campos LM, Omori CH, Braga AL, et al. Risk factors for juvenile dermatomyositis: Exposure to tobacco and air pollutants during pregnancy. Arthritis Care Res (Hoboken). 2014 Oct;66(10):1571–1575.
- Bernatsky S, Fournier M, Pineau CA, et al. Associations between ambient fine particulate levels and disease activity in patients with systemic lupus erythematosus (SLE). Environ Health Persp. 2011 Jan;119(1):45–49.
- Vidotto JP, Pereira LA, Braga AL, et al. Atmospheric pollution: Influence on hospital admissions in paediatric rheumatic diseases. Lupus. 2012 Apr;21(5):526–533.
- Molodecky NA, Panaccione R, Ghosh S, et al. Challenges associated with identifying the environmental determinants of the inflammatory bowel diseases. Inflamm Bowel Dis. 2011 Aug;17(8):1792–1799.