
The recent Ebola epidemic in West Africa (more than 5,500 dead), with infection now having been carried to countries in other continents, has prompted a need for clinicians as well as public health officials to better understand the pathobiology of Ebola infection.
Although much remains to be learned about how to best manage patients infected with Ebola viruses, studies of the virus in tissue culture, mice, nonhuman primates (NHPs) and affected humans over the past two decades have yielded informative insights into viral pathogenesis and host responses to the virus. These studies are of interest not only to the clinical virologist but also to clinical immunologists in that they provide evidence for significant dysregulation of innate immune responses during Ebola infection that correlate with mortality. Indeed, the ability of the host to effectively attenuate initial inflammatory responses to Ebola infection appears to correlate with favorable outcomes. As such, whether in the presence or absence of effective antiviral therapies, intervention opportunities currently at our disposal may be useful for the supportive care of Ebola patients.
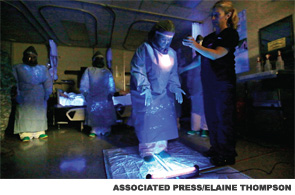
Ebola Virus
The structure and functional constituents of the five known Ebola virus subtypes have been well summarized in recent reviews.1-3 Ebola viruses are enveloped, single-stranded (-)RNA viruses (see Table 1). The lipid bilayer capsules are heavily glycosylated, which facilitates attachment to a variety of cell-surface membranes. The genome encodes for seven structural elements: nucleoprotein (NP), glycoprotein (GP), RNA-dependent RNA polymerase (L) and four structural proteins termed VP24, VP30, VP35 and VP40. In addition to facilitating viral replication, several of the gene products contribute to immune evasion by inhibiting activation of antiviral interferon genes in the host (VP24 and VP35) or coding for secretion of decoy targets (truncated sGP) that engender non-neutralizing antibodies.4
Herein, we focus on the clinical features and immunologic dysregulation observed during infection with Ebola and other hemorrhagic fever viruses, with emphasis on attributes shared with an entity familiar to rheumatologists in which similar clinical and laboratory findings are manifest—macrophage activation syndrome (MAS).
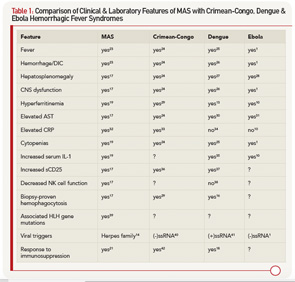
Pathogenesis & Clinical/Laboratory Features
Following the transmission of Ebola virus, studies in NHPs indicate the virus initially infects differentiated dendritic cells and macrophages.5 Initial clinical manifestations in infected individuals who become symptomatic are nonspecific, with fever, headache, myalgia and enteritis, appearing anywhere between two and 21 days.6 With progression to stages of greater severity, Ebola virus is subsequently detected in endothelial cells, hepatocytes and other tissues, with clinical and laboratory features that are very similar to what is observed in other hemorrhagic fever viruses (see Table 1).7 Liver dysfunction, including AST and ALT elevations, is quite frequent and may be accompanied by jaundice. Although leukocytosis may occur early, this is frequently followed by the development of significant lymphopenia and neutropenia. Profound coagulopathy with hypofibrinogenemia, d-dimer elevations, LDH elevations and thrombocytopenia develop as preterminal events in association with mucosal hemorrhage and multi-organ failure.
Host Immunologic Response
Within hours of Ebola binding to macrophages, even prior to evidence of viral replication, there is significant macrophage activation and release of proinflammatory cytokines.8,9 These in vitro observations correlate with significant measured serum elevations in macrophage-derived cytokines, including IL-1β, TNF, IL-6, IL-15, IL-16 and IL-8, as well as chemokines, including MIP-1, MCP-1, M-CSF and IP-10, that are observed during the early stages of infection.10 Although mRNA expression studies confirm evidence of eventual T cell activation, elevations in cytokines specific to T cell activation, such as IL-12 or IL-17, are typically not observed in the early stages of infection. Noteworthy is the apparent suppression of interferon responses that is mediated by two viral proteins, VP24, which blocks nuclear localization of interferon triggered transcription factors, and VP35, which inhibits or inactivates several type-I interferon regulatory factors (IRF-3, IRF-7).4 The ability of the virus to attenuate antiviral interferon responses may, therefore, contribute to the establishment and rapid dissemination of infection in the host.
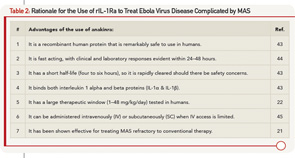
It is instructive and important to recognize that while the symptomatic attack rate in Ebola virus is very high, not all individuals who acquire the virus develop symptoms.11 In prospective studies of family members having close contact with symptomatic Ebola virus disease patients, a number of individuals mount effective IgM and IgG responses to viral proteins in the context of confirmed viremia with genotype identical virus, yet do not develop symptoms. In these individuals, high levels of proinflammatory macrophage-derived cytokines, including IL-1β (see Table 1), TNF, IL-6, MCP and MIP-1α/β, are observed early, within five to seven days of the initial contact. However, this occurs in temporal association with a robust antiinflammatory response with measured high concentrations of IL-1Ra, sTNFRI/sTNFRII, IL-10 and cortisol, with rapid decline in the levels of the proinflammatory cytokines to normal two to three days after the initial rise. Although T cell-derived cytokines were not detected in the plasma of asymptomatic infected individuals, increased IL-2, IL-4, IL-12, IFNγ, Fas, FasL and perforin mRNA expression occurs and peaks nine to 16 days after exposure. These observations are consistent with the development of a cytotoxic CD8 T cell response required for the killing of Ebola-infected cells, as well as CD4 T helper cell responses that likely promote the noted rise in Ebola-specific IgG1 and IgG3 antibody levels.
For the majority of infected individuals who become symptomatic with Ebola, the persistence of and high levels of macrophage-derived cytokines, as well as serum ferritin, an established marker of sustained macrophage activation, have been shown to correlate with disease severity and mortality.10 Whether or not, and to what extent, a sustained and unregulated innate inflammatory response impairs the development of effective humoral and cytotoxic responses required to ultimately clear the virus remains uncertain. However, the contribution of sustained high levels of TNF to lymphoid apoptosis may account for the observed lymphopenia and associated absence of effective T cell-dependent humoral and cytotoxic T cell responses in the advanced stages of severe infection.
A Case for MAS
Significant hyperferritinemia (levels >20,000 ng/mL), as well as the clinical and laboratory features observed in severe Ebola and the other viral hemorrhagic fevers, are strikingly similar to what is commonly observed in patients who develop MAS in the setting of a variety of underlying illnesses, including rheumatic diseases (e.g., systemic juvenile idiopathic arthritis, systemic lupus erythematosus, adult-onset Still disease), immunodeficiency states, infection with herpes viruses (e.g., EBV, CMV, HHV-6, HSV) (see Table 1) or T cell leukemia.14 MAS is a frequently fatal complication of these disorders that results from a proinflammatory cytokine storm.14 Along these lines of thought, MAS (sometimes referred to as secondary hemophagocytic lymphohistiocytosis, or HLH) and hemophagocytosis noted on pathology specimens have already been associated with other hemorrhagic fever viruses, including Crimean-Congo and Dengue.15,16 Indeed, elevated serum ferritin levels have been reported in Dengue and associated with the severity of MAS/HLH manifestations in Crimean-Congo fever.12,13 It is, therefore, highly plausible to consider Ebola hemorrhagic fever as another likely trigger of MAS/HLH.
In prospective studies of family members having close contact with symptomatic Ebola virus disease patients, a number of individuals mount effective IgM & IgG responses to viral proteins in the context of confirmed viremia with genotype identical virus, yet do not develop symptoms.
Potential Therapy for Ebola Hemorrhagic Fever
Primary HLH, resulting from homozygous defects in lymphocyte cytolytic pathway genes, presents in infancy and is uniformly fatal unless treated with an aggressive etoposide-based protocol (HLH-2004) followed by bone marrow transplantation.14 By contrast, secondary HLH/MAS, typically presenting beyond infancy, is often treated with immunosuppressive therapy such as high-dose corticosteroids and cyclosporine A.17 Indeed, immunosuppression has successfully treated Dengue hemorrhagic fever (see Table 1).18 Recently, targeted cytokine inhibition has been shown to successfully treat HLH/MAS.19 Blockade of the proinflammatory cytokines, IL-1 and IL-6, have both proven beneficial in treating MAS/cytokine storm.17,20
In particular, blockade with the drug anakinra, a recombinant human IL-1 receptor antagonist (rhIL-1Ra), has been successfully used to treat refractory cases of MAS.21 There are several reasons to strongly consider the use of rhIL-1Ra as MAS therapy for Ebola hemorrhagic fever (see Table 2). Although rhIL-1Ra does not have a formal indication for use in MAS, it has been tested in more than 6,000 people with a variety of disorders, including those with FDA approval (e.g. rheumatoid arthritis), and as a recombinant human protein that binds excess IL-1 (both IL-1β and IL-1β), it has been shown to be remarkably safe. This includes doses as high as 48 mg/kg/day in sepsis trials where it did not have adverse effects compared with placebo.22 When rhIL-1Ra is effective in treating MAS, the clinical (e.g., fever) and laboratory (e.g., ferritin, AST) improvements are easily tracked and are notable within 24–48 hours. In addition, anakinra has a very short half-life (four to six hours), so even if it was deleterious, it can be stopped and out of the system in very short order. Lastly, it can be given subcutaneously in situations in which intravenous access is limited. Desperate times, an ever-expanding epidemic in which mortality from infection approaches 70%, call for desperate measures. Sometimes, a paradigm shift is in order. In this case, treating the overly exuberant host immune response to the pathogen may provide an opportunity to decrease mortality.
Summary
An epidemic of Ebola virus disease is killing dozens of people daily in Western Africa with no end in sight, and—except passive immunity—there is no effective therapy or vaccine currently available. Like other hemorrhagic fever viruses (Dengue and Crimean-Congo), Ebola infection demonstrates clinical and laboratory features strikingly reminiscent of MAS. MAS secondary to infection can be treated with immunosuppression, dampening the detrimental host immune response. Perhaps, the safest and most rapidly effective therapy for MAS in a time of uncertainty is rhIL-1Ra. It will likely benefit patients with Ebola hemorrhagic fever, but if it does not, it is likely to be safe in the short term and not harmful.
Heaven forbid either of the authors become ill with Ebola virus, but if so, please begin rhIL‑1Ra therapy, and call for a rheumatology consult.

W. Winn Chatham, MD, is professor of medicine and the Louis W. Heck Clinical Scholar in Rheumatology in the Division of Clinical Immunology and Rheumatology at the University of Alabama at Birmingham, where he serves as the associate division director for clinical services. In addition to coordinating clinical and translational studies in the UAB Lupus Clinic, Dr. Chatham has clinical interests in disorders of macrophage activation and immunodeficiency-associated autoimmunity.

Randy Q. Cron, MD, PhD, is professor of pediatrics and medicine and the inaugural holder of the Arthritis Foundation, Alabama chapter–endowed chair in Pediatric Rheumatology, and he is the division director and the fellowship director of Pediatric Rheumatology at the University of Alabama at Birmingham. His laboratory studies CD4 T cell transcription as it relates to systemic lupus erythematosus and to HIV-1 infection, as well as genetic mutations associated with macrophage activation syndrome that lead to decreased cytolytic function of lymphocytes.
Disclosures
Dr. Cron has previously served as a consultant to Swedish Orphan Biovitrum, receiving less than $5,000.
References
- Ansari AA. Clinical features and pathobiology of Ebolavirus infection. J Autoimmun. 2014 Sep 23.
- Carroll SA, Towner JS, Sealy TK, et al. Molecular evolution of viruses of the family Filoviridae based on 97 whole-genome sequences. J Virol. Mar 2013;87(5):2608–2616.
- Stahelin RV. Membrane binding and bending in Ebola VP40 assembly and egress. Front Microbiol. 2014;5:300.
- Wong G, Kobinger GP, Qiu X. Characterization of host immune responses in Ebola virus infections. Expert Rev Clin Immunol. 2014 Jun;10(6):781–790.
- Geisbert TW, Young HA, Jahrling PB, et al. Pathogenesis of Ebola hemorrhagic fever in primate models: Evidence that hemorrhage is not a direct effect of virus-induced cytolysis of endothelial cells. Am J Pathol. 2003 Dec;163(6):2371–2382.
- Feldmann H, Geisbert TW. Ebola haemorrhagic fever. Lancet. 2011 Mar 5;377(9768):849–862.
- Feldmann H. Ebola–a growing threat? N Engl J Med. Oct 9 2014;371(15):1375–1378.
- Stroher U, West E, Bugany H, et al. Infection and activation of monocytes by Marburg and Ebola viruses. J Virol. 2001 Nov;75(22):11025–11033.
- Wahl-Jensen V, Kurz S, Feldmann F, et al. Ebola virion attachment and entry into human macrophages profoundly effects early cellular gene expression. PLoS Negl Trop Dis. 2011 Oct;5(10):e1359.
- McElroy AK, Erickson BR, Flietstra TD, et al. Ebola hemorrhagic fever: Novel biomarker correlates of clinical outcome. J Infect Dis. 2014 Aug 15;210(4):558–566.
- Leroy EM, Baize S, Debre P, Lansoud-Soukate J, Mavoungou E. Early immune responses accompanying human asymptomatic Ebola infections. Clin Exp Immunol. 2001 Jun;124(3):453–460.
- Barut S, Dincer F, Sahin I, et al. Increased serum ferritin levels in patients with Crimean-Congo hemorrhagic fever: Can it be a new severity criterion? Int J Infect Dis. 2010 Jan;14(1):e50–e54.
- van de Weg CA, Huits RM, Pannuti CS, et al. Hyperferritinaemia in dengue virus infected patients is associated with immune activation and coagulation disturbances. PLoS Negl Trop Dis. 2014 Oct;8(10):e3214.
- Janka GE. Familial and acquired hemophagocytic lymphohistiocytosis. Annu Rev Med. 2012;63:233–246.
- Karti SS, Odabasi Z, Korten V, et al. Crimean-Congo hemorrhagic fever in Turkey. Emerg Infect Dis. 2004 Aug;10(8):1379–1384.
- Tan LH, Lum LC, Omar SF, Kan FK. Hemophagocytosis in dengue: comprehensive report of six cases. J Clin Virol. 2012 Sep;55(1):79–82.
- Ravelli A, Grom AA, Behrens EM, et al. Macrophage activation syndrome as part of systemic juvenile idiopathic arthritis: Diagnosis, genetics, pathophysiology and treatment. Genes Immun. 2012 Jun;13(4):289–298.
- Pal P, Giri PP, Ramanan AV. Dengue associated hemophagocytic lymphohistiocytosis: A case series. Indian Pediatr. 2014 Jun;51(6):496–497.
- Schulert GS, Grom AA. Macrophage activation syndrome and cytokine-directed therapies. Best Pract Res Clin Rheumatol. 2014 Apr;28(2):277–292.
- Maude SL, Frey N, Shaw PA, et al. Chimeric antigen receptor T cells for sustained remissions in leukemia. N Engl J Med. 2014 Oct 16;371(16):1507–1517.
- Miettunen PM, Narendran A, Jayanthan A, et al. Successful treatment of severe paediatric rheumatic disease-associated macrophage activation syndrome with interleukin-1 inhibition following conventional immunosuppressive therapy: Case series with 12 patients. Rheumatology (Oxford). 2011 Feb;50(2):417–419.
- Opal SM, Fisher CJ Jr., Dhainaut JF, et al. Confirmatory interleukin-1 receptor antagonist trial in severe sepsis: A phase III, randomized, double-blind, placebo-controlled, multicenter trial. The Interleukin-1 Receptor Antagonist Sepsis Investigator Group. Crit Care Med. 1997 Jul;25(7):1115–1124.
- Weaver LK, Behrens EM. Hyperinflammation, rather than hemophagocytosis, is the common link between macrophage activation syndrome and hemophagocytic lymphohistiocytosis. Curr Opin Rheumatol. 2014 Sep;26(5):562–569.
- Bakir M, Ugurlu M, Dokuzoguz B, et al. Crimean-Congo haemorrhagic fever outbreak in Middle Anatolia: A multicentre study of clinical features and outcome measures. J Med Microbiol. 2005 Apr;54(Pt 4):385–389.
- Yacoub S, Wills B. Predicting outcome from dengue. BMC Med. 2014 Sep 4;12(1):147.
- Rathakrishnan A, Klekamp B, Wang SM, et al. Clinical and immunological markers of dengue progression in a study cohort from a hyperendemic area in Malaysia. PLoS One. 2014;9(3):e92021.
- Santhosh VR, Patil PG, Srinath MG, et al. Sonography in the diagnosis and assessment of dengue fever. J Clin Imaging Sci. 2014;4:14.
- Bwaka MA, Bonnet MJ, Calain P, et al. Ebola hemorrhagic fever in Kikwit, Democratic Republic of the Congo: Clinical observations in 103 patients. J Infect Dis. Feb 1999;179 Suppl 1:S1–S7.
- Bicakci Z, Tavil B, Tezer H, et al. Hemophagocytosis in a case with Crimean-Congo hemorrhagic fever and an overview of possible pathogenesis with current evidence. Turk J Pediatr. 2013 May–Jun;55(3):344–348.
- Ahmed A, Alvi AH, Butt A, et al. Assessment of dengue fever severity through liver function tests. J Coll Physicians Surg Pak. 2014 Sep;24(9):640–644.
- Rollin PE, Bausch DG, Sanchez A. Blood chemistry measurements and D-Dimer levels associated with fatal and nonfatal outcomes in humans infected with Sudan Ebola virus. J Infect Dis. 2007 Nov 15;196 Suppl 2:S364–371.
- Shimizu M, Yokoyama T, Yamada K, et al. Distinct cytokine profiles of systemic-onset juvenile idiopathic arthritis-associated macrophage activation syndrome with particular emphasis on the role of interleukin-18 in its pathogenesis. Rheumatology (Oxford). 2010 Sep;49(9):1645–1653.
- Yilmaz G, Koksal I, Topbas M, et al. The effectiveness of routine laboratory findings in determining disease severity in patients with Crimean-Congo hemorrhagic fever: Severity prediction criteria. J Clin Virol. Apr 2010;47(4):361–365.
- Ho TS, Wang SM, Lin YS, et al. Clinical and laboratory predictive markers for acute dengue infection. J Biomed Sci. 2013;20:75.
- Suharti C, van Gorp EC, Setiati TE, et al. The role of cytokines in activation of coagulation and fibrinolysis in dengue shock syndrome. Thromb Haemost. Jan 2002;87(1):42–46.
- Deveci K, Oflaz MB, Sancakdar E, et al. Evaluation of the serum levels of soluble IL-2 receptor and endothelin-1 in children with Crimean-Congo hemorrhagic fever. APMIS. Jul 2014;122(7):643–647.
- Valero N, Larreal Y, Espina LM, et al. Elevated levels of interleukin-2 receptor and intercellular adhesion molecule 1 in sera from a Venezuelan cohort of patients with dengue. Arch Virol. 2008;153(1):199–203.
- Homchampa P, Sarasombath S, Suvatte V, et al. Natural killer cells in dengue hemorrhagic fever/dengue shock syndrome. Asian Pac J Allergy Immunol. Dec 1988;6(2):95–102.
- Zhang M, Behrens EM, Atkinson TP, et al. Genetic defects in cytolysis in macrophage activation syndrome. Curr Rheumatol Rep. 2014;16(9):439–446.
- Walter CT, Barr JN. Recent advances in the molecular and cellular biology of bunyaviruses. J Gen Virol. Nov 2011;92(Pt 11):2467–2484.
- Rodriguez-Roche R, Gould EA. Understanding the dengue viruses and progress towards their control. Biomed Res Int. 2013;2013:690835.
- Dilber E, Cakir M, Erduran E, et al. High-dose methylprednisolone in children with Crimean-Congo haemorrhagic fever. Trop Doct. Jan 2010;40(1):27–30.
- Granowitz EV, Porat R, Mier JW, et al. Pharmacokinetics, safety and immunomodulatory effects of human recombinant interleukin-1 receptor antagonist in healthy humans. Cytokine. Sep 1992;4(5):353–360.
- Behrens EM, Kreiger PA, Cherian S, et al. Interleukin 1 receptor antagonist to treat cytophagic histiocytic panniculitis with secondary hemophagocytic lymphohistiocytosis. J Rheumatol. Oct 2006;33(10):2081–2084.
- Yang BB, Baughman S, Sullivan JT. Pharmacokinetics of anakinra in subjects with different levels of renal function. Clin Pharmacol Ther. Jul 2003;74(1):85–94.