The Case
An 11-year-old Caucasian female with juvenile dermatomyositis (JDMS) presented to our clinic with a worsening rash and increased proximal muscle weakness. Three years prior to this visit, the patient had been diagnosed with JDMS based on clinical presentation, elevated muscle enzymes, and characteristic findings on muscle biopsy and electromyography. She was successfully managed with chronic oral corticosteroids and weekly oral methotrexate.
Six months prior to her initial visit in our clinic, the patient developed a recurrence of her heliotrope rash and Gottron’s papules of her hands and knees. She also experienced worsening proximal muscle weakness, with difficulty brushing her hair, walking up and down stairs and standing up from a seated position. Magnetic resonance imaging (MRI) of her thighs revealed diffuse T2 signal abnormalities bilaterally, consistent with myositis. Tests with abnormal results included a creatine kinase of 383 U/L (60–230 U/L), aldolase of 13.2 U/L (1.2–11.8 U/L), lactate dehydrogenase of 1,042 U/L (340–700 U/L), neopterin of 71.4 nmol/L (2–10 nmol/L), and immunoglobulin G (IgG) of 1,810 mg/dl (524–1,403 mg/dL).
In view of these findings, the patient was started on a new treatment regimen that consisted of subcutaneous methotrexate at a higher dose, oral corticosteroids, and azathioprine. Nevertheless, the patient continued to have a rash and progressive muscle weakness. A decision was therefore made to start treatment with intravenous pulse corticosteroids and monthly high-dose intravenous immunoglobulin (IVIg) infusions. Immediately following the start of this treatment, the patient noticed marked improvement in both her rash and strength. Her muscle enzymes normalized after three months of IVIg therapy, and she was able to ride a bicycle for the first time in two years. Her cutaneous findings subjectively improved after four months of IVIg therapy, and her neopterin level normalized after 12 months. She maintained remission on monthly high-dose IVIg infusions and stable methotrexate dosing with continued tapering of the oral corticosteroids. Her IVIg therapy was discontinued after 14 total infusions, and her JDMS has remained quiescent on subcutaneous methotrexate and hydroxychloroquine.
Introduction
IVIg is a highly purified globulin preparation obtained from the pooled plasma of thousands of healthy donors. Although initially given as replacement therapy for patients with primary and secondary immunodeficiency states, IVIg has proven to be effective in the treatment of various autoimmune and inflammatory disorders. This success has led to a dramatic increase in the use of IVIg, with its use as an antiinflammatory agent now vastly surpassing its use in the treatment of immunodeficiencies. In fact, approximately 70% of IVIg administered today is for immunomodulation in patients with autoimmune disease.1 Even so, the basis for the antiinflammatory activity of IVIg remains unclear. While multiple modes of action have been proposed, there may not be a single effect and the different proposed actions may not be mutually exclusive. This article will review some of these mechanisms, preceded by a brief discussion of clinically relevant aspects of IVIg therapy.
Historical Aspects
The first application of immunoglobulin therapy dates back to the 19th century when immune sera from vaccinated animals were used for prevention and treatment of tetanus, diphtheria, and other infectious diseases. Not until the 1940s were immunoglobulins isolated from human plasma and purified into the first preparations available for use. With anticipation of the United States’ entry into World War II, the National Research Council approached Dr. Edwin Cohn at Harvard University to produce a readily available blood product for the treatment of shock. Albumin was considered an ideal agent for this purpose. Dr. Cohn therefore developed a fractionation process that used cold alcohol to divide plasma proteins into subfractions, producing large amounts of albumin as well as fractions rich in immunoglobulins. Additional modifications resulted in the creation of a successful large-scale fractionation technique and the first immunoglobulin formulations. This Cohn-Oncley cold alcohol fractionation process is still used today.2
Administration of these early formulations of immunoglobulin was often complicated by systemic reactions likely due to contamination with impurities and complement activation by protein aggregates. In 1982, the World Health Organization provided standards for the manufacturing of IVIg products. According to these standards, IVIg should be derived from a pool of at least 1,000 donors. It should have minimal quantities of aggregates, but should also be at least 90% intact with maintenance of full biological functions. IVIg should contain as little IgA as possible and also be free of infectious agents.3 In 1984, the first commercial product from the Swiss Red Cross, Sandoglobulin (Sandoz/Novartis), was licensed for use in the United States. Subsequent refinements to the original Cohn cold alcohol fractionation technique, including additives to prevent IgG re-aggregation, have made newer intravenous preparations safe and well tolerated.
Not All Preparations Are Equivalent
Several preparations of IVIg are now licensed and available in the United States, and selecting the best product for a patient can be challenging (See Table 1). Product features that are important to consider include sugar content, sodium concentration, volume load, osmolality, and amount of IgA. The approaches used to minimize formation of IgG aggregates and stabilize preparations account for most of the variations in composition. Sugars, such as sorbitol, maltose, and sucrose, or amino acids are added to some formulations to prevent aggregate formation. The pH also affects stability, and at an optimum but acidic pH of 4.0–4.5, IgG molecules are present mostly as monomers and additional stabilizers are not required.4
The carbohydrate content of a preparation is particularly important for patients with diabetes mellitus, as glucose-containing products may acutely affect glycemic control. In addition, a disproportionate share of renal adverse events is associated with sucrose-containing products.3,4 Sugar and sodium content also both affect osmolality, and increased osmolality may be of concern in patients with a history of renal dysfunction, elevated blood pressure or heart failure. Lyophilized products needing reconstitution also tend to have higher osmolality than liquid formulations of IVIg. Patients who cannot tolerate increased osmolality may also not tolerate a high volume load, mostly determined by IVIg dose and product concentration. Lastly, all IVIg preparations contain a small amount of IgA, and patients with IgA deficiency may be at risk of anaphylaxis because of their production of IgE anti-IgA antibodies, particularly with prior exposure to blood products containing IgA. Anaphylaxis is rare, however, and most patients with IgA deficiency tolerate products with low IgA content.4
Safety and Infusion-Related Adverse Events
A majority of the side effects associated with IVIg are minor, self-limited, and typically related to the infusion rate (see Table 2). The most commonly reported side effects are headache, nausea, myalgia, arthralgias, urticaria, low-grade fever, chest discomfort, and tachycardia. Milder reactions can often be reduced or prevented by decreasing the speed of the infusion or temporarily discontinuing the infusion and restarting at a lower rate after symptoms resolve. If a patient continues to experience adverse reactions with treatment, a trial of premedications including antihistamines, analgesics, and rarely corticosteroids is reasonable. Switching to a different brand of product is also worth considering.3
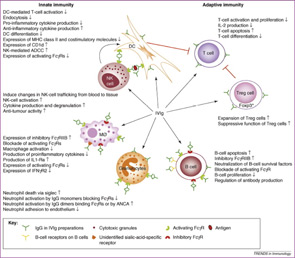
Major side effects of IVIg therapy include aseptic meningitis, acute renal failure, thrombotic complications, and, rarely, anaphylaxis. Patients with a history of migraine headaches in particular have a predisposition toward aseptic meningitis. Prehydration, slower infusion rates, and premedication with analgesics and a migraine-specific medication such as sumatriptan may help prevent this complication. Acute renal failure is thought to be due to an osmotic nephrosis leading to acute tubular necrosis. As previously mentioned, the literature estimates that 90% of cases of acute renal failure are associated with the infusion of sucrose-containing preparations of IVIg. A significant number of patients developing this complication also have other comorbid conditions, including baseline renal insufficiency, hypertension, and diabetes mellitus. Thrombotic events are likely related to increases in plasma viscosity above the physiologyic range of 285–295 mOsmol/kg. Myocardial infarction and stroke are rare and potentially fatal side effects that may be due to increases in serum viscosity. Lower-concentration solutions and slower speeds of infusion may decrease the potential for thrombotic complications. For high doses of IVIg, however, lower concentrations may demand larger volumes of fluid and providers should be careful of volume overload.3,4
Any plasma product has a potential for transmission of infection. In the 1990s, cases of acute hepatitis C were reported in patients receiving IVIg. A number of steps were then taken to reduce the risk of transmitting blood-borne pathogens, including more careful donor screening and selection, better testing of plasma donations and more effective procedures for virus inactivation. There have since been no subsequent reports of hepatitis C virus infection. Nor have there been reports of transmission of HIV, and the cold alcohol fractionation process is an effective method for the removal and inactivation of virus. Similarly, transmission risk of human prion disease has not been reported with the use of IVIg.3 Vigilance is required, however, and all patients receiving IVIg should be counseled and monitored for transmission of infections.
Clinical Applications and Indications
IVIg was first proven to be an effective immunomodulator over three decades ago, when Paul Imbach at University Children’s Hospital Basel in Switzerland reported successful treatment of acute and chronic idiopathic thrombocytopenic purpura (ITP) with this agent. In these pioneering experiments, Imbach treated 13 patients with 2 gm/kg of IVIg divided over five consecutive days, and observed a dramatic and rapid rise in platelet counts in all patients.5 At present, IVIg is FDA approved for six indications other than ITP: primary immunodeficiency disorders (PID), secondary immunodeficiency in chronic lymphocytic leukemia (CLL), pediatric HIV infection, Kawasaki disease (KD), chronic inflammatory demyelinating polyneuropathy (CIDP), and prevention of graft-versus-host disease and infection following adult hematopoietic cell transplantation.3,6 None of the licensed products in the U.S. has approval for all indications (see Table 1). Current estimates suggest that more than 100 different conditions have been treated with IVIg.6 While the number of clinical conditions where IVIg is used continues to increase, the mechanisms of the antiinflammatory effects of high-dose IVIg remain poorly understood, limiting development of a more scientific rationale for its use and biomarkers to assess its action and guide patient selection.
Potential Mechanisms of Action
Many modes of action have been proposed for IVIg, and different mechanisms may be responsible for its antiinflammatory activity in different diseases (see Figure 1). Imbach’s initial observations led to subsequent studies to elucidate IVIg’s therapeutic efficacy in ITP, and one of the earliest hypothesized mechanisms was the functional blockade of IgG receptors (FcγR) on phagocytes. Specifically, IVIg competition for, and occupation of, FcγRs was postulated to prevent uptake and clearance of antibody-coated platelets by the reticuloendothelial system. This theory was supported by studies on the clearance of autologous radiolabeled, antibody-sensitized erythrocytes in four adults with ITP treated with high-dose IVIg. In these experiments, IVIg not only prolonged the clearance time of the radiolabeled erythrocytes, but also transiently increased the platelet counts to normal levels within four to five days.7
Many immunologists have theorized that autoimmune disorders result from aberrant function of the idiotypic network, and that IVIg contains antiidiotype antibodies that restore regulation by this pathway. Since immune responses to foreign antigens need to be self-limited, the idiotypic network has been postulated as a way to downregulate these immune responses specifically. According to this hypothesis, the variable regions of antigen receptors on T cells (TCR) and immunoglobulins contain unique amino acid sequences that determine their antigen specificity. This region is called the idiotope; all TCRs or antibodies that share an idiotope belong to the same idiotype. When a foreign antigen enters the system, one or a few clones of lymphocytes respond, and their idiotypes are expanded. Along with the expansion of particular idiotypes, antiidiotype responses are also triggered. These antiidiotypes can in turn downregulate responses by shutting off the antigen-specific or idiotype-expressing lymphocytes.1
According to this idea, antiidiotypic antibodies present in IVIg can bind to either surface immunoglobulin on B cells or circulating antibodies in plasma. IVIg can therefore ameliorate autoimmune disease by directly inhibiting autoantibody binding to its target antigen; it may also target autoreactive B-cell clones expressing or secreting the autoantibody for destruction. One disease that serves as a model for this proposed mechanism is acquired hemophilia caused by inhibitory autoantibodies to Factor VIII. Autoantibodies to Factor VIII may arise in nonhaemophilic patients with autoimmune disease, such as rheumatoid arthritis or systemic lupus erythematosus, as well as otherwise healthy individuals. Administration of high-dose IVIg in patients with acquired hemophilia can result in a rapid and prolonged depression of anti-VIIIc antibody titers. Further suggesting that antiidiotype antibodies are the active component of IVIg, subsequent studies showed that infusion of only the antigen-binding fragments of IVIg can reduce levels of the pathogenic antibody.8
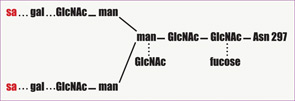
An additional way that IVIg may modulate inflammation is the accelerated degradation of pathogenic IgG by saturation of the neonatal Fc receptor (FcRn), so named because it was initially identified in the neonatal intestinal epithelium. This IgG receptor aids in the transfer of IgG from mother to fetus and also protects IgG from degradation. FcRn is known to exist in many adult tissues, including intestinal epithelium, muscle, and skin. In states of hypergammaglobulinemia, (e.g., following high-dose IVIg), the FcRn is presumably saturated and the catabolism rate of the pathogenic antibody is accelerated. In murine models of autoimmune-mediated skin blistering diseases (e.g., bullous pemphigoid) administration of high-dose IVIg drastically reduces pathogenic IgG levels and prevents blistering. In contrast, mice deficient in FcRn are resistant to the development of blistering in this model and administration of high-dose IVIg does not confer any additional protective effect.9
The inhibitory properties of IVIg may also result from its ability to scavenge active complement proteins, and studies have shown that high levels of IgG can inhibit the uptake of C3b and C4b onto the surface of sensitized guinea pig and human erythrocytes and prevent complement-mediated tissue damage. It is thought that IVIg binds the activated complement components C3b and C4b and therefore prevents their deposition onto target surfaces.10,11 A disease model in which IVIg convincingly modulates complement is dermatomyositis (DM). Dermatomyositis is an inflammatory myopathy mediated by the deposition of complement and formation of the C5-9 membrane attack complex (MAC) on intramuscular capillaries, leading to loss of capillaries and muscle ischemia and necrosis. Marinos Dalakas and colleagues from the National Institute of Neurological Disorders and Stroke evaluated whether high-dose IVIg can inhibit complement activity in a series of patients with DM treated with IVIg. They confirmed that IVIg reduces C3 uptake onto antibody sensitized erythrocytes.12 In addition, patients clinically improved and repeat muscle biopsies revealed disappearance of C3 and MAC deposits and improvement of the muscle architecture with an increase in muscle fiber size and number of muscle capillaries.12,13
In recent years, there has been a revolution in our understanding of the antiinflammatory effect of IVIg, with the important discovery that IVIg can be separated into extensively sialylated and nonsialylated IgG fractions. Essentially all of the effector functions of IgG, including the Fc-binding activity and the protective activity against pathogens, reside in the nonsialylated fraction. The sialylated fraction, comprising only 5% or less of the IgG pool, appears to contain all of the antiinflammatory activity. In murine models of inflammatory arthritis, administration of high-dose IVIg protects mice from developing joint inflammation. IVIg pretreated with neuraminidase to remove the terminal sialic acid residues, however, does not exhibit antiinflammatory activity. Conversely, in the experiments in mice, a sialic acid–enriched IVIg preparation showed a 10-fold enhancement in protection so that near equivalent protection was obtained at one-tenth the dose of unfractionated IVIg.14 An attractive possibility for developing new and more potent treatment would be a recombinant IVIg preparation that is composed of hypersialylated IgG molecules. (See Figure 2)
The antiinflammatory activity of the sialylated IgG is now thought to be mediated by binding of this type of IgG to SIGN-R1 (specific ICAM-3–grabbing nonintegrin-related 1) that is expressed on splenic macrophages in mice, or the human orthologue DC-SIGN (dendritic cell–specific intercellular adhesion molecule-3-grabbing non-integrin) expressed on dendritic cells. The binding of sialylated IgG to DC-SIGN on dendritic cells is reported to trigger an antiinflammatory pathway that ultimately results in enhanced expression of inhibitory FcγRs on effector macrophages. The upregulation of inhibitory FcγRs aids in the attenuation of inflammation.15
Other proposed mechanisms of action of IVIg include modulation of adhesion molecules expressed on various cell surfaces, suppression of cytokine expression, enhancement of regulatory T-cell function, and inhibition of dendritic cell maturation and function.1 High-dose IVIg may also upregulate expression of the inhibitory FcγRIIB, thereby exerting a negative feedback inhibition on antibody production.16 Thus, studies suggest that IVIg affects many steps in the immune response, and the critically important step may differ in various disease states.
Conclusion
Significant progress has been made in understanding the antiinflammatory effects of IVIg in the treatment of autoimmune and chronic inflammatory disorders. But, with the expansion of the number of clinical situations in which high doses of IVIg are effective, the risk of worldwide shortages of this agent also increases. A review of the literature highlights the need for more well-designed, controlled studies. Such studies are imperative to identify the clinical applications where IVIg is truly therapeutic. These studies will also provide more insight into the mechanisms of immune modulation. Further research is also needed to develop a molecularly engineered preparation of a recombinant IVIg to optimize its therapeutic efficacy and reduce the required dosage and thereby potential for shortage.
Disclosure
Dr. Frank is a consultant for CSL Behring. Dr. Wu is a fellow in the divisions of rheumatology and allergy/immunology, and Dr. Frank is the Samuel L. Katz Professor of Pediatrics, division of allergy/immunology, department of pediatrics, Duke University Medical Center, Durham, N.C.
References
- Ballow M. The IgG molecule as a biological immune response modifier: Mechanisms of action of intravenous immune serum globulin in autoimmune and inflammatory disorders. J Allergy Clin Immunol. 2011;127:315-323.
- Berger M. A history of immune globulin therapy from the Harvard crash program to monoclonal antibodies. Curr Allergy Asthma Rep. 2002;2:368-378.
- Ballow M. Safety of IGIV therapy and infusion-related adverse events. Immunol Res. 2007;38:122-132.
- Gelfand EW. Differences between IGIV products: Impact on clinical outcome. Int Immunpharmacol. 2006;6:592-599.
- Imbach P, d’Apuzzo V, Hirt A, et al. High-dose intravenous immunoglobulin for idiopathic thrombocytopenic purpura in childhood. Lancet. 1981;1:1228-1231.
- Nimmerjahn F, Ravetch JV. Antiinflammatory actions of intravenous immunoglobulin. Annu Rev Immunol. 2008;26:513-533.
- Fehr J, Hofmann V, Kappeler U. Transient reversal of thrombocytopenia in idiopathic thrombocytopenic purpura by high-dose intravenous immunoglobulin. N Eng J Med. 1982;306:1254-1258.
- Sultan Y, Masionneuve P, Kazatchkine MD, et al. Anti-idiotypic suppression of autoantibodies to factor VIII (antihaemophilic factor) by high-dose intravenous gammaglobulin. Lancet. 1984;2:765-768.
- Li N, Zhao M, Hilario-Vargas J, et al. Complete FcRn dependence for intravenous Ig therapy in autoimmune skin blistering diseases. J Clin Invest. 2005;115:3440-3450.
- Basta M, Langlois PF, Marques M, et al. High-dose intravenous immunoglobulin modifies complement-mediated in vivo clearance. Blood. 1989;74:326-333.
- Basta M, Fries LF, Frank MM. High doses of intravenous Ig inhibit in vitro uptake of C4 fragments onto sensitized erythrocytes. Blood. 1991;77:376-380.
- Basta M, Dalakas MC. High-dose intravenous immunoglobulin exerts its beneficial effect in patients with dermatomyositis by blocking endomysial deposition of activated complement fragments. J Clin Invest. 1994;94:1729-1735.
- Dalakas MC, Illa I, Dambrosia JM, et al. A controlled trial of high-dose intravenous immune globulin infusions as treatment for dermatomyositis. N Eng J Med. 1993;329:1993-2000.
- Kaneko Y, Nimmerjahn F, Ravetch JV. Antiinflammatory activity of immunoglobulin G resulting from Fc sialylation. Science. 2006;313:670-673.
- Anthony RM, Wermeling F, Karlsson MC, et al. Identification of a receptor required for the antiinflammatory activity of IVIg. Proc Natl Acad Sci USA. 2008;105:19571-19578.
- Samuelsson A, Towers TL, Ravetch JV. Antiinflammatory activity of IVIg mediated through the inhibitory Fc receptor. Science. 2001;291:484-486.