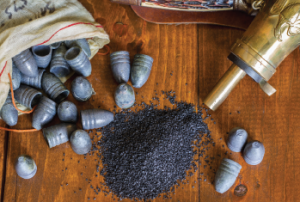
Matt Cuda / shutterstock.com
Black powder was initially developed in 9th century China, by Taoists searching for the philosopher’s stone, which fans of Harry Potter will remember is the talisman that grants eternal life. The Chinese name for black powder literally translates as fire medicine. The chemical composition of black powder was first recorded in China during the 11th century and prominently featured saltpeter, or potassium nitrate, which was a key component of many medicinal compounds at that time.1
Soon after the composition of black powder was recorded, its raison d’être became apparent: You could set it on fire. Black powder is called a low explosive, because when it ignites, it decomposes slowly, and it creates a propulsive force at subsonic speeds, enough to propel a bullet without rupturing the barrel of a gun. And so the modern era of warfare was born.
But that’s not the story I want to tell.
Evolution
The same features that made black powder, also known as gunpowder, effective in munitions also made it ineffective in a field far more lucrative than warfare—mining. Its low explosive force made it ineffectual at rending rock face so miners could reach the valuable minerals that lay beneath. Gunpowder was a low explosive. What the miners needed was a high explosive.
Miners would have to wait until 1847, when an Italian chemist named Ascanio Sobrero wrote a monograph on “detonating compounds” that could be produced by combining nitric acid with a number of substances, including sugar, dextrin, lactose and glycerine. Dr. Sobrero wrote, “The results that I have just obtained prove that glycerine is capable of giving, when mixed with nitric and sulfuric acids, a substance similar to gun cotton” or nitrocellulose, which was the most powerful explosive at that time.2
Dr. Sobrero actually discouraged the commercial use of nitroglycerin. In his subsequent papers, he described characterizing the properties of nitroglycerin by using just a few drops, because the compound was so unstable.3 The problem was that commercial applications, like mining, required crates—not just drops—of nitroglycerin to be transported over long distances.
The results were predictable. In 1867, the state of California banned the transport of liquid nitroglycerin.4 Other states soon followed, and eventually heavy restrictions on the transportation of nitroglycerin became commonplace.
The Accidental Prize
This was a problem for a munitions company that had gradually morphed into a company specializing in the production of nitroglycerin for excavation and mining. Its profits in danger, Alfred Nobel & Company focused on developing a safer, more stable version of nitroglycerin. Mr. Nobel was the son of an inventor, and perhaps it is not surprising he figured out that by combining nitroglycerin with the diatomaceous earth that surrounded his factory in Hamburg, he was able to stabilize dynamite for transport.5 When combined with his other invention, the blasting cap, which allowed for remote detonation, it was the perfect explosive.
But that’s not the story I want to tell, either.
There is an expression that, “if you build a better mousetrap, the world will beat a path to your door.” Mr. Nobel had succeeded in building a better mousetrap. His brilliance may not have been in the invention of dynamite, however, but may have been in his aggressive defense of his patent for dynamite. If you wanted this particular mousetrap, you would have to buy it from him.
Gradually, this led to the accumulation of an enormous amount of wealth under a single person.
How did we get from there to the Nobel Prize? It may have been an accident.
In 1888, Alfred’s brother, Ludvig Nobel, died. A French journalist, thinking it was Alfred who had passed on, wrote, “le marchand de la mort est mort”—or “the merchant of death is dead.”6 Although he had not yet died, the description was otherwise accurate. Alfred Nobel continued to make large amounts of money from the sale of munitions, in addition to the sale of explosives. This weighed on him heavily, and it is said this headline, along with his brother’s passing and his own approaching mortality, made him think carefully about the legacy he wanted to leave behind.
It also may have been a bit of luck. Upon Alfred Nobel’s death, he left the majority of his wealth to the creation of a series of prizes to be awarded by the Royal Swedish Academy of Sciences, the Karolinska Institute, the Swedish Academy and the Parliament of Norway. But there were a few problems:
“The will of Alfred Nobel came as a complete surprise; he had discussed it with no one before his death. It was handwritten on a sheet of paper, with notes scribbled in the margin, and had never been reviewed by a lawyer, since Nobel distrusted lawyers. … They faced several problems:
- There was no Nobel Foundation, so the money had been left to no one, to nothing;
- The will was imprecise, and it was not determined even in what country the will should be probated;
- Some of the family, who were left only a small fraction of the estate, contested the will;
- The press and others criticized the will, calling it unpatriotic, because it did not single out Swedes for the prize. They also argued that it should not be used to make a few individuals wealthy and voiced other objections;
- The assets of the estate were not liquid, and in fact were in risky ventures;
- The institutions chosen to award the prizes weren’t willing to accept the responsibility.”7
In addition to all of these issues, Ragnar Sohlman and Rudolf Lilljequist, the executors of Mr. Nobel’s will, had to figure out how to liquidate and transfer Mr. Nobel’s wealth from France, where he had lived, back to Sweden, before the French government could tax the proceeds. It was their efforts, over three years, which led to the realization of the Nobel Foundation and the Nobel Prize.8
Meandering Toward Greatness
By now, it is probably obvious to you why this story is relevant to us. On Oct. 1, the 2018 Nobel Prize in Physiology or Medicine was awarded to James P. Allison, PhD, of the United States and to Tasuku Honjo, MD, PhD, of Japan for their work on cancer immunotherapy.9
Dr. Allison coined the phrase checkpoint inhibitor to describe the function of CTLA-4 on downregulating T cell function. Dr. Honjo discovered a second checkpoint, called PD-1, which is the target of nivolumab and pembrolizumab. Many forms of cancer make proteins that bind to PD-1 or CTLA-4, which prevents the malignancy from activating an immune response. By inhibiting the checkpoints, these biologic agents prevent the malignancy from inactivating T cells, leaving them free to attack.
This approach has led to a revolution in the treatment of cancer, and many patients who have malignancies previously deemed incurable have been given a reprieve by the advent of the immune checkpoint inhibitors.
But that isn’t the story I want to tell, either.
The immune checkpoint inhibitor story is well known to us, from sources ranging from the New England Journal of Medicine to The New York Times. We are involved because it turns out that malignancy and autoimmunity may be two sides of the same coin, and by abolishing one, we may induce the other.10 The story is worth telling, and indeed, has been told in the pages of this publication, and I might write more about this fascinating field at some point in the future. But not today.
Today, I want to tell you about the Nobel Prize in Chemistry, which was awarded on Oct. 3, 2018, to Frances H. Arnold, PhD, of the California institute of Technology, George P. Smith, PhD, of the University of Missouri, and Sir Gregory P. Winter of the MRC Laboratory of Molecular Biology in England, for developing the field of synthetic biology.11
Synthetic biology is the intersection of molecular biology and engineering, which involves multiple fields, including computer science, biophysics and nanotechnology. The goal of synthetic biology is to learn how to redesign biologic systems to achieve a particular purpose.
Revolutions in medicine always start with the non-medical, with discoveries that seem far afield. Because they are.
Dr. Smith developed phage display.12 Bacteriophages, as you remember from medical school, are viruses that infect bacteria. Dr. Smith learned how to use bacteriophages to characterize protein interactions with other molecules. Although the specifics of the technique are well beyond my undergraduate biochemistry degree, the basic principle is more straightforward: A DNA fragment encoding the protein of interest is inserted into the phage genome, so the protein is expressed on the surface of the virus. Various proteins or other targets of interest are attached to the bottom of plates and put into contact with the phage. If the phage protein sticks to the target, you have identified a significant interaction. If you started with multiple DNA fragments, you can then harvest the phage to identify the relevant DNA fragment.
Dr. Winter used the technique to solve problems—in this case, the problem of the monoclonal antibody. At the time, monoclonal antibodies existed, but were derived from mice and were, therefore, highly immunogenic. The development of the half-mouse, half-human chimeric monoclonal antibody was a workaround that led to the development of infliximab. Dr. Winter used the phage display technique to identify a fully human anti-tumor necrosis factor monoclonal antibody, which led to D2E7, which you may know by its other name, adalimumab.13
Since then, this technique has been used to develop belimumab and a host of monoclonal antibodies under development for the treatment of a variety of diseases. As our understanding of the pathogenesis of our diseases deepens, this technique will increase in relevance to us, and to our patients.
As you may have guessed by now, the point of this discursive story is less to discuss the Nobel Prize in Chemistry and more to illustrate the meandering, non-
linear path of discovery. The funding agency that initially supported Dr. Smith’s work on bacteriophages could not have possibly foreseen that his work would lead to the drug I now give to my patients with rheumatoid arthritis. The true story goes back even further: While studying infectious diseases in India, it was Ernest Hankin who discovered a compound in the Ganges that seemed to kill cholera. Félix d’Hérelle concluded this compound must be a “virus parasitic on bacteria,” which he named a bacteriophage.14 Without Mr. Hankin’s work in India, adalimumab and belimumab might not exist today.
The story of Alfred Nobel and the Nobel Prize shows the role serendipity plays in events we now take for granted. It must have been providence that placed Mr. Nobel’s factories near a deposit of diatomaceous earth, which was the perfect stabilizing agent for nitroglycerin. Also, none of the original clients of Alfred Nobel & Company could possibly have suspected the money they had paid Mr. Nobel for gunpowder and dynamite would eventually fund a prize for chemistry or medicine or peace.
Serendipity and discovery, of course, will take you only so far. Someone must have paid for Mr. Hankin to travel to India. Similarly, without the fortune produced with dynamite, Mr. Nobel’s noble intentions would have been for naught. As research agencies continue to tighten their belts, it has not escaped anyone’s notice that we now tend to fund incrementalism. Shrinking budgets have made the National Institutes of Health risk-adverse, so more than ever, funding dollars go to support established investigators who are building upon their previous work.15
It’s hard not to bet on a sure thing, but it is equally hard to wonder what amazing advances we will never make because of this overly cautious approach. There is a non-linear path from discovery to invention, from the theoretical to the practical. Revolutions in medicine always start with the non-medical, with discoveries that seem far afield. Because they are. For-profit entities will never fund this sort of research; epoch-making discoveries are the province of basic science research that is publicly funded. Only by seeing the sciences as a national priority can we hope to fuel the next, explosive advance.
Philip Seo, MD, MHS, is an associate professor of medicine at the Johns Hopkins University School of Medicine, Baltimore. He is director of both the Johns Hopkins Vasculitis Center and the Johns Hopkins Rheumatology Fellowship Program.
References
- Johnson NG. Explosive. Encyclopaedia Britannica. 2015 May 7.
- Sobrero A. Sur plusieur composés détonants produits avec l’acide nitrique et le sucre, la dextrine, la lactine, la mannite et la glycérine. Comptes rendus. 1847;24:247–248.
- Sobrero A. Sopra alcuni nuovi composti fulminanti ottenuti col mezzo dell’azione dell’acido nitrico sulle sostante organiche vegetali. Memorie della Reale Accademia delle Scienze di Torino (2nd series). 1849;10:195–201.
- Liquid nitroglycerin and the transcontinental railroad. American Experience, Season 15. 2013 Jan 27.
- Drahl C. About dynamite, the reason we have Nobel prizes. Forbes. 2016 Oct 1.
- Schultz C. Blame sloppy journalism for the Nobel prizes. Smithsonian.com. 2013 Oct 9.
- Brau CA. Shepherd VL. Ragnar Sohlman and the will of Alfred Nobel. Class notes for Honors 182, the Nobel Prize Winners in Science, Vanderbilt University, 1/16/2005; material from a variety of sources including Ragnar Sohlman, The Legacy of Alfred Nobel (The Bodley Head Ltd. London, 1983)..
- Ragnar Sohlman. The Nobel prize.
- Grady D. 2018 Nobel Prize in Medicine awarded to 2 cancer immunotherapy researchers. The New York Times. 2018 Oct 1.
- Cappelli LC, Shah AA, Bingham CO 3rd. Immune-related adverse effects of cancer immunotherapy—implications for rheumatology. Rheum Dis Clin North Am. 2017 Feb;43(1):65–78.
- Chang C. Use of evolution to design molecules nets Nobel Prize in Chemistry for 3 scientists. The New York Times. 2018 Oct 3.
- Pande J, Szewczyk MM, Grover AK. Phage display: Concept, innovations, applications and future. Biotechnol Adv. 2010 Nov–Dec;28(6):849–858.
- Barrera P, Joosten LA, den Broeder AA, et al. Effects of treatment with a fully human anti-tumor necrosis factor alpha monoclonal antibody on the local and systemic homeostasis of interleukin 1 and TNF alpha in patients with rheumatoid arthritis. Ann Rheum Dis. 2001 Jul;60(7):660–669.
- Fruciano E. Phage as an antimicrobial agent: d’Herelle’s heretical theories and their role in the decline of phage prophylaxis in the West. Can J Infect Dis Med Microbiol. 2007 Jan;18(1):19–26.
- Carroll AE. Why the medical research grant system could be costing us great ideas. The New York Times. 2018 Jun 18.