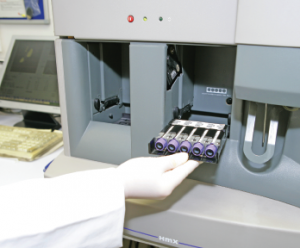
A technician inserts a rack of blood samples into the HmX flow cytometry machine.
BSIP/Science Source
SAN DIEGO—In his doctoral work, Sean Bendall, PhD, assistant professor of pathology and a researcher at Stanford University in Palo Alto, Calif., worked on protein identification and embryonic stem cell biology. That required examining the characteristics of cells—lots of cells. He was struck by how inefficient the process was.
“The issue was, every experiment I did required me to switch up 1 million, 2 million, 3 million cells and [pull] them out of the well to put them on the machine to get my list of proteins, and then I had to go back and figure out—one cell at a time because I was dealing with stem cells—what those proteins were doing,” he said in a talk at the 2017 ACR/ARHP Annual Meeting Nov. 3–8.
The traditional process of single-cell analysis is full of hurdles: The more information you try to get out of an assay, the more the signals can bleed into one another, and compensating for this interference sacrifices sensitivity and quantitative power. And you may have only one tube available for analysis. Plus, it’s time consuming.
Maybe there’s a better way, he thought.
Since then, his work has focused on ways of raising the multiplexing level of assays; that is, how to ask more questions of cells and obtain answers more quickly. Called “mass cytometry,” it’s an evolving approach that holds the promise of providing answers—in rheumatology and other fields—to questions researchers could barely even ask before, let alone answer.
The basic mass cytometry approach, developed several years ago but with new tweaks and applications all the time, involved changing from a fluorescence-based assay to one based on mass spectrometry, and using nonbiological elements as the cell characteristic reporters, such as europium and holmium. These elements are found in cell phone components and microchips, but not in the bloodstream, so there’s little interference from the biological background, Dr. Bendall said. “You’re only limited by how pure you can get the different reporters,” he said.
Once all the cells are stained, they’re diluted, then sprayed as single-cell droplets into a mass spectrometer—Dr. Bendall describes it as spraying a clear liquid into a box. Then the cells are converted into a cloud of ions, and then into an elemental measurement for each cell. The resulting readout shows the levels of the items researchers want to know about.
The traditional fluorescence-based approach, he said, “doesn’t leave a lot of room to look at the things I think a lot of researchers are generally interested in, which is cell behavior. Is the cell dividing? Is it dying? Is it signaling? Is my drug doing something to it? Do the cells die more, or signal more or less?”
In one of the first mass cytometry applications, researchers captured a snapshot of all the hematopoietic immune cells in healthy human bone marrow—at the same time, in one experiment.
They created an algorithm called “SPADE,” with cluster size representing the number of cells in the cluster, and with colors representing the marker researchers wanted to display. They used only about half of their available markers on phenotypic traits, which left plenty of markers to track behavioral characteristics.1
“You can then use this model to compare these different conditions and see how they perturb behavior of the cells, and in what cells it’s going on,” Dr. Bendall said.
We’ve got the ability to measure millions of cells per day, & now we’re pushing up to 50 parameters for every cell in our experiment. —Dr. Bendall
He underscored the element of discovery possible with the approach.
“Even though we were doing a completely targeted assay—we knew what we were measuring ahead of time—we were still generating de novo information,” he said. “I didn’t need to know exactly where the activity was going to be, because I was capturing and organizing all the cells at the same time.”
More recently, researchers have used the approach to track the behavior of chimeric antigen receptor T cells, in which immune cells are engineered and re-infused and then expand in the body in an attempt to better attack cancers such as lymphoma. They’ve developed a tool to model this process and assign each cell a division ID, and can use the dilution of dyes to follow the cells’ division because as the cells divide, the dyes dilute. And, they can track the cells’ characteristics along the way.
“We’ve got the ability to measure millions of cells per day, and now we’re pushing up to 50 parameters for every cell in our experiment,” Dr. Bendall said.
For instance, by tracking CD-69, a commonly observed cell-activation marker, researchers could tell the cells chose one phenotype over another very early in the process.
“Almost all [cell] decisions are being made in terms where the cells are going, and what they’re becoming, prior to the first division,” Dr. Bendall said. Researchers have also found the cells seem able to be manipulated without much loss in terms of expansion.
Information like this could prove powerful in developing and refining therapy, Dr. Bendall said.
“The idea is that we can build these models up where we can uncouple time and differentiation and division, look at what pathways are on and off, and when they’re on and off,” he said. “And [then we] start thinking about smart ways we can steer the cells into the phenotypes we want them to be.”
Thomas R. Collins is a freelance writer living in South Florida.
Reference
- Qiu P, Simonds EF, Bendall SC, et al. Extracting a cellular hierarchy from high-dimensional cytometry data with SPADE. Nat Biotechnol. 2011 Oct 2;29(10):886-891.