Checking blood levels of commonly used disease-modifying anti-rheumatic drugs (DMARDs) has gained widespread attention in the rheumatology community, even resulting in a recent guidance document from EULAR for biologics.1 Although a highly useful tool, drug level measurement in rheumatology is not without challenges; many of our drugs violate the basic principles of pharmacology that we learned in medical or nursing school. For example, biologic drugs can have non-linear kinetics; mycophenolate undergoes enterohepatic recirculation; and hydroxychloroquine (HCQ) is highly variable in drug pharmacokinetics between patients. If this sounds intimidating, don’t worry. This tutorial covers the basics of therapeutic drug monitoring (aka the art of checking drug levels) and provides practical guidance for both clinicians and researchers.
The Need for Therapeutic Drug Monitoring
Historically, therapeutic drug monitoring was best used for drugs that had a narrow therapeutic index—that is, drugs for which toxicity could occur with small changes in dosing or individual alterations in drug pharmacokinetics. However, we’ve since learned that pharmacokinetics for our commonly used small molecule and biologic DMARDs vary widely among patients with rheumatic diseases. This wide variability in pharmacokinetics can occur due to a patient’s genetics, which alter drug metabolizing enzymes or drug transporters; the effects of a disease, such as hypoalbuminemia from lupus nephritis or impaired gastric motility from systemic sclerosis; and differences in body composition; among other causes. Practically speaking, there is a growing recognition that no one-size-fits-all approach exists to dosing for DMARDs.
Drug level measurement is one tool that looks downstream from dosage to better relate drug exposure to clinical response, and can be used to individualize a patient’s dosage. Additionally, drug levels can be used to identify medication non-adherence and allow for counseling interventions to improve patient outcomes.2
Although drug level measurements can guide clinical management, the correct interpretation of drug levels requires at least three crucial pieces of information:
- The timing since the last dosage was taken;
- The dosage; and
- The number of doses taken (e.g., adherence, attainment of steady state).
Step-by-Step Guide to Interpreting Drug Levels
Step 1: Timing Is Everything
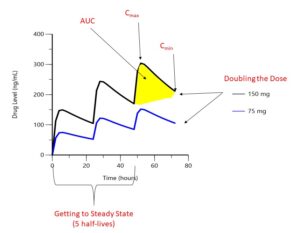
FIGURE 1: Drug levels over time. Hypothetical drug administered orally every 24 hours. (Click to enlarge.)
The first step to interpreting a drug level is finding out when your patient took their most recent dosage. As shown in Figure 1, drug concentrations change significantly between the highest concentrations (Cmax or peak) and its lowest (Cmin or trough). The area under the curve (AUC) represents the total concentration of drug present over a given period of time. For an intravenous (IV) drug, maximum concentrations occur at the end of the IV infusion, whereas for an oral or subcutaneous drug, maximum concentrations occur when the process of absorption equals the process of elimination, which although unique to each drug, is typically hours after the dose is taken for small molecules and days for biologic drugs. Trough concentrations are typically measured at the end of the dosing interval, right before the next dosage is due. When a patient first begins a medication, drug levels will accumulate with each dosage until steady state is reached, which occurs after five drug half-lives; so it is important to know how long your patient has been taking their medication.
Checking random drug levels is generally only useful for drugs with very long half-lives and low within-day variability. Azathioprine metabolites (half-life of approximately five days) and most subcutaneous biologic drugs (apart from anakinra) fall into this category.3
Checking random drug levels of other medications for the purpose of identifying medication adherence can sometimes be useful when concentrations are at the extremes. For example, undetectable drug levels often imply some degree of medication nonadherence. Many recent publications, particularly those studying HCQ, have relied on random drug levels. However, recent data suggest that HCQ levels change by about 30% within a day, significantly limiting the interpretation of these studies and highlighting the need to study trough levels.4 Mycophenolic acid can undergo absorption more than once (enterohepatic recirculation), resulting in erratic time vs. concentration profiles and the need to measure AUC as opposed to a peak, trough or random concentration.
Although asking patients to take their medication at a specific time is ideal so you can measure a timed sample (e.g., peak or trough) when they see you in clinic, patients can find this difficult, logistically speaking. However, by asking your patient when they took their last dosage, it is possible, in most cases, to measure a random drug level and ballpark where they are in the dosing interval—near the peak, trough or somewhere in between. This strikes a reasonable balance between practicality and interpretability in most cases.
Step 2: Dose Matters
Most DMARDs (except certain biologics, particularly at low doses) follow linear pharmacokinetics, meaning dose is directly proportional to drug exposure (Cmax, Cmin, AUC). For example, a patient receiving 150 mg of a medication would be expected to have twice the Cmax as somebody receiving 75 mg of the same medication (see Figure 1). Therefore, when comparing a medication blood level for your patient to a target level from the literature, it is important to consider whether the dose your patient is receiving is similar to doses in the research study. This also means that drug level thresholds used to identify medication non-adherence are also dose specific.
A quick note on weight-based dosing: A common pitfall in our community is assuming that normalizing dosage based on weight (e.g., 5 mg/kg of HCQ or 2 mg/kg of azathioprine) will ensure a diverse group of patients will all achieve comparable drug levels. Unfortunately, studies have shown a very poor correlation between weight-based dosing and drug levels for HCQ (R2=0.03)5 and azathioprine/6-MP (r=0.17).6 The reasons for the poor correlation are complex, but weight-based dosing assumes a linear relationship between body weight and dose, whereas in reality drug clearance often follows an allometric (power) relationship. However, in children, weight-based dosing is still important for many drugs.
Step 3: Compare Apples to Apples
Whenever we check drug concentrations in a patient, we need to be able to compare this level with some target from the literature to know whether or not it is in a range that is efficacious, toxic or associated with non-adherence. As seen in steps 1 and 2 of this tutorial, it is crucial to compare apples to apples—drug levels at the same dose and taken at the same time, noting whether the sample was drawn at steady state. A few other reasons may explain why drug levels differ across studies, including the assay used to detect the drug, the units of measure (e.g., ng/mL vs. μg/mL) and the matrix/sample the drug is measured in (e.g., whole blood, serum or plasma).
Table 1 summarizes the typical matrix and assay used for various DMARDs. For reference, 1 ng/mL=0.001 mg/L=0.001 μg/mL.
The majority of drug levels are measured in plasma or serum, which generally have similar performance characteristics. However, certain drugs, such as HCQ, partition into red blood cells, meaning whole blood levels are much higher than in plasma or serum and cannot be directly compared. For HCQ, whole blood levels are more precise and are generally preferred for non-pregnant patients.7 Additionally, different assays are used to measure drug levels, and the assays may or may not correlate well with one another. Most small molecule DMARDs can be detected using high-performance liquid chromatography (HPLC). However, assay techniques for biologic drugs are variable and perform differently in the presence of anti-drug antibodies.1
Step 4 (The Last Step): It’s Your Patient
The final step to interpreting a drug level is to individualize the results to the patient in front of you. Individual patients may have drug levels that deviate from expected for a variety of reasons, including medication non-adherence, physiologic alterations in drug pharmacokinetics (e.g., genetics, extremes of body weight, and effects of their disease on organ function), the presence of anti-drug antibodies and drug-drug interactions, among others. Individual interpretation is drug specific and requires a basic understanding of the drug’s pharmacology. For example, HCQ is excreted renally, and blood levels are higher in patients with significant renal disease. As a second example, mycophenolate mofetil drug levels are reduced in the presence of a protonpump inhibitor and if administered with magnesium or aluminum hydroxide antacids.
Although outside the scope of this tutorial, our future practices will likely be heavily influenced by the rapidly growing field of pharmacogenomics and pharmacokinetics modeling, which may help us individualize drug dosing by identifying which patients require dosage adjustments and providing the bedside clinical decision tools to change dose.
Putting It All Together
For DMARDs in rheumatology, the two most common reasons to check drug levels are to gauge adherence to medication and to identify whether patients are achieving a therapeutic or toxicity threshold requiring a dosage adjustment, especially in states of altered states of physiology (e.g., renal dysfunction, gastric bypass) or in the presence of an anti-drug antibody. Additional reasons may include exploring reasons for primary or secondary non-response, or to guide tapering decisions. Table 1 provides a high-level (and nonexhaustive) summary of published drug level targets across several biologic and non-biologic DMARDs; more extensive summaries have been published elsewhere.7
Identifying medication non-adherence is crucial because it avoids the common scenario where a non-adherent patient may appear to fail multiple different treatments and be labeled as having treatment-refractory disease. Moreover, counseling interventions have been shown to improve adherence and result in higher systemic drug levels.2 In general, a good case can be made to check drug levels when a patient is not responding to treatment, although some clinicians check drug levels routinely as part of their practice. As outlined in Table 1, HCQ has the best data with medication non-adherence. However, due to high variability in HCQ pharmacokinetics, an algorithm has been proposed to optimally relate HCQ blood levels with the number of missed doses.4
Additional data are needed before most DMARDs can be routinely dose-adjusted to achieve target levels based on efficacy or toxicity, with the strongest data for tacrolimus (not covered in this tutorial) and biologic drugs. For example, a recent randomized controlled trial found that patient’s achieving a 3–8 μg/mL trough level of infliximab had higher rates of remission compared to usual care.9 Algorithms have been proposed for tumor necrosis factor (TNF) inhibitors to help guide the decision on whether non-responders should have a dosage increase, be switched to a biologic of the same drug class or be switched to a different drug class.10 A clinical trial in adults with systemic lupus erythematosus and HCQ blood levels between 100 and 750 ng/mL were randomized to usual care vs. dose escalation to a blood HCQ level of ≥1,000 ng/mL; the study found no significant difference in flare rates over seven months.11 However, there were observed changes in medication adherence, and most patients randomized to dose escalation did not achieve the target HCQ level. In subgroup analyses, patients who consistently maintained HCQ levels ≥1,000 ng/mL had significantly fewer flares (excluding flares within one month of randomization). For mycophenolate, ongoing clinical trials are evaluating the efficacy of AUC guided dosing vs. standard dosing in children with SLE.
Avoiding Common Pitfalls: Interpreting Blood Levels for Efficacy
As noted in Table 1, a variety of therapeutic target levels have been proposed for various drugs, some of which vary widely. How can we make sense of different target drug levels for the same condition?
First, it is important to recall that the gold standard for determining target drug levels (and dosage) is through a dedicated pharmacokinetic/pharmacodynamic study. These studies are specifically designed to minimize background medications, standardize follow-up and generally enroll a narrow patient phenotype. In contrast, many existing studies in Table 1 are observational, with patients on a variety of concomitant, background medications (often with no prohibition of dose changes), have a variety of baseline disease characteristics and, often, have different lengths of follow-up. Therefore, many of these target drug levels are highly context specific—that is, the optimal level in that specific population and set of circumstances.
Additionally, different target levels could exist for a reason we already discussed—differences in timing across studies. Using random HCQ blood levels for example, it would be possible for a patient in a study to be classified as having both therapeutic and non-therapeutic levels, depending entirely on when the measurement was taken. Therefore, random blood level targets from the literature should be interpreted cautiously for most drugs.
Lastly, recall that a drug may exert different effects (and treat different conditions) based on its level. Aspirin is a classic example: The dosage of aspirin needed for the anti-platelet effect is substantially less than that needed for the anti-inflammatory effect. By extension, it is possible that the exposure-response relationship for one disease or manifestation (e.g., arthritis vs. lupus nephritis) may be different.
Conclusions
In closing, therapeutic drug monitoring is a rapidly growing field in rheumatology, with the potential to transform clinical practice by individualizing dosing and distinguishing treatment failure arising from lack of response vs. medication non-adherence. To properly interpret drug levels, rheumatologists must carefully consider the principles outlined in this tutorial. To optimize future efficacy studies, researchers must prospectively collect precise information on dosage, timing and adherence; ensure disease homogeneity; control for background medications; and ensure standardized follow-up with outcomes that reflect the drug’s response.
Stephen Balevic, MD, PhD, RhMSUS, is an adult and pediatric rheumatologist and clinical pharmacologist at Duke University, Durham, N.C., where he is an associate director of pharmacometrics at the Duke Clinical Research Institute. He received his doctorate in pharmaceutical sciences from the University of North Carolina Eshelman School of Pharmacy, Chapel Hill.
References
- Krieckaert CL, van Tubergen A, Gehin JE, et al. EULAR points to consider for therapeutic drug monitoring of biopharmaceuticals in inflammatory rheumatic and musculoskeletal diseases. Ann Rheum Dis. 2023 Jan;82(1):65–73.
- Durcan L, Clarke WA, Magder LS, Petri M. Hydroxychloroquine blood levels in systemic lupus erythematosus: Clarifying dosing controversies and improving adherence. J Rheumatol. 2015;42(11):2092–2097.
- Derijks LJ, Gilissen LP, Engels LG, et al. Pharmacokinetics of 6-thioguanine in patients with inflammatory bowel disease. Ther Drug Monit. 2006 Feb;28(1):45–50.
- Balevic et al. Interpreting hydroxychloroquine blood levels for medication non-adherence: A pharmacokinetic study. Manuscript under review.
- Balevic SJ, Randell R, Weiner D, et al. Pharmacokinetics of hydroxychloroquine in paediatric lupus: data from a novel, direct-to-family clinical trial. Lupus Sci Med. 2022 Nov; 9(1): e000811.
- Morales A, Salguti S, Miao CL, Lewis JD. Relationship between 6-mercaptopurine dose and 6-thioguanine nucleotide levels in patients with inflammatory bowel disease. Inflamm Bowel Dis. 2007 Apr;13(4):380–385.
- Balevic SJ, Weiner D, Clowse MEB, et al. Hydroxychloroquine PK and exposure-response in pregnancies with lupus: The importance of adherence for neonatal outcomes. Lupus Sci Med. 2022 Jan;9(1):e000602.
- Balevic SJ, Sagcal-Gironella ACP. Precision medicine: Towards individualized dosing in pediatric rheumatology. Rheum Dis Clin North Am. 2022 Feb;48(1):305–330.
- Syversen SW, Jorgensen KK, Goll GL, et al. Effect of therapeutic drug monitoring vs standard therapy during maintenance infliximab therapy on disease control in patients with immune-mediated inflammatory diseases: A randomized clinical trial. JAMA. 2021 Dec;326(23):2375–2384.
- Vincent FB, Morand EF, Murphy K, et al. Antidrug antibodies (ADAb) to tumour necrosis factor (TNF)-specific neutralising agents in chronic inflammatory diseases: A real issue, a clinical perspective. Ann Rheum Dis. 2013; 72(2):165–178.
- Costedoat-Chalumeau N, Galicier L, Aumaître O, et al. Hydroxychloroquine in systemic lupus erythematosus: results of a French multicentre controlled trial (PLUS Study). Ann Rheum Dis. 2013 Nov;72(11):1786–1792.
- Chen DY, Chen YM, Tsai WC, et al. Significant associations of antidrug antibody levels with serum drug trough levels and therapeutic response of adalimumab and etanercept treatment in rheumatoid arthritis. Ann Rheum Dis. 2015 Mar;74(3):e16.
- Pouw MF, Krieckaert CL, Nurmohamed MT, et al. Key findings towards optimising adalimumab treatment: The concentrationeffect curve. Ann Rheum Dis. 2015;74(3): 513–518.
- Sanmarti R, Inciarte-Mundo J, Estrada-Alarcon P, et al. Towards optimal cut-off trough levels of adalimumab and etanercept for a good therapeutic response in rheumatoid arthritis. Results of the INMUNOREMAR study. Ann Rheum Dis. 2015 Aug;74(8):e42.
- Vande Casteele N, Herfarth H, Katz J, Falck-Ytter Y, Singh S. American Gastroenterological Association Institute technical review on the role of therapeutic drug monitoring in the management of inflammatory bowel diseases. Gastroenterology. 2017 Sep;153(3):835–857 e836.
- Daien CI, Daien V, Parussini E, et al. Etanercept concentration in patients with rheumatoid arthritis and its potential influence on treatment decisions: A pilot study. J Rheumatol. 2012 Aug;39(8):1533–1538.
- Frances C, Cosnes A, Duhaut P, et al. Low blood concentration of hydroxychloroquine in patients with refractory cutaneous lupus erythematosus: A French multicenter prospective study. Arch Dermatol. 2012 Apr; 148(4):479–484.
- Costedoat-Chalumeau N, Houssiau F, Izmirly P, et al. A prospective international study on adherence to treatment in 305 patients with flaring SLE: Assessment by drug levels and self-administered questionnaires. Clin Pharmacol Ther. 2019 Aug;106(2):374–382.
- Ting TV, Kudalkar D, Nelson S, et al. Usefulness of cellular text messaging for improving adherence among adolescents and young adults with systemic lupus erythematosus. J Rheumatol. 2012 Jan;39(1):174–179.
- Garg S, Unnithan R, Hansen KE, et al. Clinical significance of monitoring hydroxychloroquine levels in patients with systemic lupus erythematosus: A systematic review and metaanalysis. Arthritis Care Res (Hoboken). 2021 May;73(5):707–716.
- Zahr N, Urien S, Funck-Brentano C, et al. Evaluation of hydroxychloroquine blood concentrations and effects in childhood-onset systemic lupus erythematosus. Pharmaceuticals (Basel). 2021 Mar;14(3):273.
- Petri M, Elkhalifa M, Li J, et al. Hydroxychloroquine blood levels predict hydroxychloroquine retinopathy. Arthritis Rheumatol. 2020 Mar;72(3):448–453.
- Neumann I, Fuhrmann H, Fang IF, et al. Association between mycophenolic acid 12-h trough levels and clinical endpoints in patients with autoimmune disease on mycophenolate mofetil. Nephrol Dial Transplant. 2008 Nov;23(11):3514–3520.
- Woillard JB, Bader-Meunier B, Salomon R, et al. Pharmacokinetics of mycophenolate mofetil in children with lupus and clinical findings in favour of therapeutic drug monitoring. Br J Clin Pharmacol. 2014 Oct;78(4):867–876.
- Lertdumrongluk P, Somparn P, Kittanamongkolchai W, et al. Pharmacokinetics of mycophenolic acid in severe lupus nephritis. Kidney Int. 2010 Aug;78(4):389–395.
- Zahr N, Arnaud L, Marquet P, et al. Mycophenolic acid area under the curve correlates with disease activity in lupus patients treated with mycophenolate mofetil. Arthritis Rheum. 2010 Jul;62(7):2047–2054.
- Godron-Dubrasquet A, Woillard JB, Decramer S, et al. Mycophenolic acid area under the concentration-time curve is associated with therapeutic response in childhood-onset lupus nephritis. Pediatr Nephrol. 2021 Jul;36(2):341–347.
- Chen Y, Sun L, Xu H, et al. PK/PD Study of mycophenolate mofetil in children with systemic lupus erythematosus to inform model-based precision dosing. Front Pharmacol. 2020 Dec;11:605060.
- Sagcal-Gironella AC, Fukuda T, Wiers K, et al. Pharmacokinetics and pharmacodynamics of mycophenolic acid and their relation to response to therapy of childhood-onset systemic lupus erythematosus. Semin Arthritis Rheum. 2011 Feb;40(4):307–313.
- Askanase AD, Wallace DJ, Weisman MH, et al. Use of pharmacogenetics, enzymatic phenotyping, and metabolite monitoring to guide treatment with azathioprine in patients with systemic lupus erythematosus. J Rheumatol. 2009 Jan;36(1):89–95.